Main group azido compounds are chemical compounds consisting of azide, N3 bonded to a main group element.
Azido compounds are often shock sensitive. Their sensitivity correlates with the amount of ionic or covalent character the azide-element bond has, with ionic character being far more stable than covalent character. Compounds such as sodium azide – which has ionic character – tend to be less sensitive, Such compounds are relevant to high-energy-density matter. although sodium azide is, ironically, the principal gas-forming component of air bags. It is the most important azide from a commercial perspective. The other commercially important azide is the lead derivative, which is of interest because of its explosive properties.
Synthesis

Azido compounds compounds can often be prepared by salt metathesis between sodium azide or silver azide and the main group chloride. Another route involves the reactions hydrazoic acid HN3 with main group compounds containing basic ligands (alkyls, alkoxides, amides, etc.). Yet another route entails the reaction of trimethylsilyl azide with the main group fluoride, a method that exploits the stability and volatility of trimethylsilyl fluoride.
Group 1 (alkali metal)
Sodium azide is prepared by the reaction of sodium amide with nitrous oxide. This route is known as the "Wislicenus process":
- 2 NaNH2 + N2O → NaN3 + NaOH + NH3
Hundreds of tons of azides are prepared in this way annually. KN3 is prepared by treating potassium carbonate with hydrazoic acid. lithium and rubidium azide can be prepared by treating solutions of their respective sulfates with barium azide.
- Ba(N3)2 + Li2SO4 → 2 LiN3 + BaSO4
Group 2 (alkaline earth)
Barium azide, Ba(N3)2, precipitates from upon addition of sodium azide to an aqueous solution of barium bromide.
Group 13

Boron triazide, B(N3)3, was first prepared by the addition of diborane to an ethereal solution of HN3 . The compound is relatively volatile and undergoes explosive decomposition above -35°C. In contrast, aluminum azide Al(N3)3 is relatively stable and will only deflagrate in a match test. It decomposes hydrolytically within minutes when exposed to atmospheric moisture. Al(N3)3 has some synthetic applications: when generated in situ, it react with β-unsaturated cyano esters to form tetrazoles in bulk scale. Gas phase reactions of AlMe3 and HN3 have been reported to form Al(N3)3. However, at room temperature, this compound decomposes to AlN2 and AlN leading to the suggestion that Al(N3)3 can be used to prepare AlN.
Owing to the interest in GaN as a semiconductor, group 13 azido chemistry is dominated by the gallium azides. Na is a polymer. This compound gives donor stabilized gallium triazides Ga(N3)3Lm which upon heating decompose to the polymeric ∞ and produces GaN after detonation. Ga(N3)3 can readily be analyzed as its tetraphosphonium salt 2. The increased ionicity of the azido ligands and the presence of the two large counterions which diminish shock propagation make the compound significantly less sensitive.
The indium azide In(N3)3 can be prepared via the fluoride exchange route. Tetraphosphonium salt 3 is also known.
Thallium (I) azide TlN3 is shock sensitive but less so than related mercury or lead azides. TlN3 is one of the few azides that melts before it explodes. Tetraphenylphosphonium salt 3 has been crystallized.
Group 14
Diazido- and triazidomethane can be prepared via simple nucleophilic substitution of methylene chloride or bromoform respectively with the azide ion on quaternary ammonia resin. Solutions greater than 70% purity of diazidomethane have a tendency to explode with any minor mechanical disturbance such as pipetting. Tetraazidomethane C(N3)4 cannot be prepared from carbontetrahalides and instead can be obtained via a reaction of trichloroacetonitrile Cl3CCN with NaN3 albeit in relatively low yields. Yields can be significantly improved by reacting triazidomethylium hexachloroantimonate with sodium or lithium azide LiN3; this route carries a high risk of accidental detonation. In general, pure tetraazidomethane should be avoided, and even solutions should not be handled manually. The compound can explode randomly at any time without apparent provocation and a singular drop is capable of shattering glass and vacuum Dewars.
Si(N3)4 can be prepared from SiCl4 and NaN3. This method, however, also produces various silicon chloroazides which can be avoided by prolonging reaction times. Additionally, unlike the boron and aluminum azides, Si(N3)4 cannot be obtained via the reaction between SiH4 and HN3. The hexaazidosicalte salt 2 adopts an octahedral molecular geometry, a very rare case of silicon in an N6 environment.
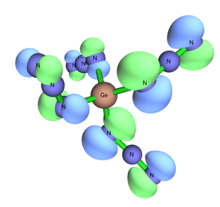
Ge(N3)4 has not been confirmed. The hexaazido germanate Na2 has been crystallized. The presence of a weakly coordinating counterion such as tetraphenylarsonium enables the formation of low valent germanates from the trichloride anion to form the germanium (II) that will not convert to the germanium (IV) upon further exposure to NaN3, but the low valent salts are very prone to oxidation.
Adducts of Sn(N3)4 are known with various ancillary ligands. Reactions with SnCl4 and NaN3 lead s to the hexaazide Na2Sn(N3)6. The salt is only slightly water sensitive and deflagrates in a flame test. Azides of Sn(II) exist in the form of salts of . Like the analogous germanates, they are very sensitive compounds. They tend to dimerize.
Lead azide is one of the most studied azides owing to its use as a primary explosive. Uniquely it is the only group 14 azide that is more prevalent in its divalent Pb form. The α, β, γ, and ∂ polymorphs exist but the α form is the only one that finds extensive technical applications. Homoleptic azides of Pb(IV) exist but like the tin versions Pb(N3)4 is not a stable compound, and attempts to synthesize it from PbO2 and HN3 form red needles that quickly explode and decompose to Pb(N3)2. The compound can however be isolated as the ions with large organic cations to yield a nonexplosive compound.
Group 15
In the general sense, azides of group 15 elements tend to resemble their chlorides but with higher volatility and less thermostability. Nitrogen rich compounds such as N(N3)3 have not yet been made.
Phosphorus triazide P(N3)3 is prepared by the reaction of NaN3 and PCl3. The existence of P(N3)5 has not yet been confirmed. Phosphorus(V) azides are restricted to from PCl3 or the sodium salt Na from PCl5.
As(N3)3 can be prepared from the fluoride exchange route, and the resulting crystal structure has EO bridging of two of the azido groups, giving a coordination number of 7 and an infinite zig-zag chain structure. However, the solution state N NMR of As(N3)3 confirms that this compounds are in fact monomeric in solution, and the lone pair of arsenic is calculated to be sterically active as evidenced by the optimized gas phase geometry and contour plot of the total electron density. Unlike phosphorus, the parent arsenic(V) azide has been isolated as As(N3)5 and exists as a yellow liquid. The entire series of arsenic azido ions have been reported, , , and . The cationic species have the shortest As-N and Nβ-Nγ distance whereas the anionic ones tend to have much longer As-N distances and therefore partially explains why the cationic compounds tend to be much more explosive.
The antimony(III) azide Sb(N3)3 is prepared in a similar manner to the arsenic one and has a similar structure with the exception that all three of azido ligands are participating in EO bridging and produce a highly symmetrical sheet. Sb(N5)5 exists as a highly unstable compound and cannot be handled at ambient temperatures without explosion. The series of antimony azide salts , , and are known and have similar trends to the arsenic ones.
Binary bismuth azides remained elusive until 2010 when clean Bi(N3)3 was isolated using the fluoride exchange route. The series of bismuth (III) ions , , and have been synthesized and structurally characterized. The bismuth lone pair is sterically active in all of the ions. In the solid state, bismuth structures tend to differ widely from their lighter group 15 counterparts since bismuth can accommodate larger coordination numbers, and the structures are based on Bi2N2 parallelogram with a coordination number of 8. Attempts at making Bi(V) compounds result in reduction to Bi(III) by N3.
Group 16
Oxygen and sulfur diazides have not been confirmed. sulfuryl azide SO2(N3)2 has been fully characterized.
The series of binary selenium azides Se(N3)4, , and have been prepared via the fluoride exchange route. The neutral Se(N3)4 detonate even at -64°Cwithout provocation. Therefore, solid state characterization is restricted to the ions, and show that the azido groups have strong covalent character. The ion crystalizes with S6 symmetry. Apparently the lone pair is not sterically active.
The tellurium (IV) azides , Te(N3)4, , and were prepared by reduction of the TeF6 via the fluoride exchange route. Te(N3)4 is a very sensitive compound, but related salts are less shock sensitive. Unlike the above selenium salt, the lone pair in the tellurium dianion is sterically active and therefore forces a distorted pseudo pentagonal bipyramidal structure.
Group 17
The four halogen azides are known: FN3, ClN3, BrN3, and IN3. The gas phase reaction between dilute F2 and HN3 produces FN3. Chlorine azide, also a gas, can be produced from passing chlorine gas through a solution of NaN3. Chlorine azide tends to explode spontaneously even at reduced temperatures. Bromine azide is a liquid but is also treacherous. BrN3 hydrolyzes readily. Similarly, iodine azide, also moisture sensitive, can be made from ICl and solid AgN3. However, since AgN3 can only be handled safely when moist, drying agent is normally added to the reaction mixture to prevent hydrolysis of the final product. Both BrN3 and IN3 find use in synthesis a convenient way to make azidiridines and azirines. The chlorine, bromine, and iodine azides have been characterized in the solid state.
See also
References
- ^ Harry David Fair; Raymond F. Walker, eds. (1977). Physics and chemistry of the inorganic azides. New York: Plenum Press. ISBN 0-306-37076-X. OCLC 2985662.
- ^ Portius, Peter; Davis, Martin (2013-03-01). "Recent developments in the chemistry of homoleptic azido complexes of the main group elements". Coordination Chemistry Reviews. Recent Developments in Main Group Chemistry. 257 (5): 1011–1025. doi:10.1016/j.ccr.2012.09.019. ISSN 0010-8545.
- Haiges, Ralf; Boatz, Jerry A.; Christe, Karl O. (2010-10-18). "The Syntheses and Structure of the Vanadium(IV) and Vanadium(V) Binary Azides V(N3)4, [V(N3)6]2−, and [V(N3)6]−". Angewandte Chemie International Edition. 49 (43): 8008–8012. doi:10.1002/anie.200906537. PMID 20715219.
- ^ Haiges, Ralf; Boatz, Jerry A.; Williams, Jodi M.; Christe, Karl O. (2011-09-12). "Preparation and Characterization of the Binary Group 13 Azides M(N3)3 and M(N3)3⋅CH3CN (M=Ga, In, Tl), [Ga(N3)5]2−, and [M(N3)6]3− (M=In, Tl)". Angewandte Chemie International Edition. 50 (38): 8828–8833. doi:10.1002/anie.201103101. PMID 21766406.
- Zhou, Jing; Zhang, Junlin; Wang, Bozhou; Qiu, Lili; Xu, Ruoqian; Sheremetev, Aleksei B. (2022). "Recent synthetic efforts towards high energy density materials: How to design high-performance energetic structures?". FirePhysChem. 2 (2): 83–139. Bibcode:2022FPhCh...2...83Z. doi:10.1016/j.fpc.2021.09.005. S2CID 244214466.
- ^ Jobelius, Horst H.; Scharff, Hans-Dieter (2000). "Hydrazoic Acid and Azides". Ullmann's Encyclopedia of Industrial Chemistry. Wiley-VCH. doi:10.1002/14356007.a13_193. ISBN 9783527306732.
- P. W. Schenk "Alkali Azides from Carbonates" in Handbook of Preparative Inorganic Chemistry, 2nd Ed. Edited by G. Brauer, Academic Press, 1963, NY. Vol. 1. p. 475.
- H. D. Fair; R. F. Walker, eds. (1977). Physics and Chemistry of the Inorganic Azides. Energetic Materials. Vol. 1. New York and London: Plenum Press. ISBN 9781489950093.
- Fraenk, Wolfgang; Habereder, Tassilo; Hammerl, Anton; Klapötke, Thomas M.; Krumm, Burkhard; Mayer, Peter; Nöth, Heinrich; Warchhold, Marcus (2001). "Highly Energetic Tetraazidoborate Anion and Boron Triazide Adducts". Inorganic Chemistry. 40 (6): 1334–1340. doi:10.1021/ic001119b. PMID 11300838.
- Wiberg, Egon; Michaud, Horst (1954-07-01). "Notizen: Zur Kenntnis eines Bortriazids B(N3)3". Zeitschrift für Naturforschung B. 9 (7): 497–499. doi:10.1515/znb-1954-0715. ISSN 1865-7117. S2CID 96674767.
- Wagner, Eugene R. (1973). "Reaction of aluminum azide with cyanoesters. Preparation of tetrazolo[1,5-c]pyrimidin-5(6H)-one and tetrazolo[1,5-c]quinazolin-5(6H)-one". The Journal of Organic Chemistry. 38 (17): 2976–2981. doi:10.1021/jo00957a012. ISSN 0022-3263.
- Linnen, C. J.; Macks, D. E.; Coombe, R. D. (1997-02-01). "Synthesis of Al(N3)3 and the Deposition of AlN Thin Films". The Journal of Physical Chemistry B. 101 (9): 1602–1608. doi:10.1021/jp9625873. ISSN 1520-6106.
- ^ Sussek, Harald; Stowasser, Frank; Pritzkow, Hans; Fischer, Roland A. (2000). <455::aid-ejic455>3.0.co;2-e "Tetraazido Complexes of Aluminium, Gallium, and Indium". European Journal of Inorganic Chemistry. 2000 (3): 455–461. doi:10.1002/(sici)1099-0682(199903)2000:3<455::aid-ejic455>3.0.co;2-e. ISSN 1434-1948.
- Frank, Alissa C.; Stowasser, Frank; Sussek, Harald; Pritzkow, Hans; Miskys, Claudio R.; Ambacher, Oliver; Giersig, Michael; Fischer, Roland A. (1998). "Detonations of Gallium Azides: A Simple Route to Hexagonal GaN Nanocrystals". Journal of the American Chemical Society. 120 (14): 3512–3513. Bibcode:1998JAChS.120.3512F. doi:10.1021/ja9738438.
- Fischer, Ronald A.; Miehr, Alexander; Herdtweck, Eberhardt; Mattner, Michael R.; Ambacher, Oliver; Metzger, Thomas; Born, Eberhard; Weinkauf, Sevil; Pulham, Colin R.; Parsons, Simon (1996). "Triazidogallium and Derivatives: New Precursors to Thin Films and Nanoparticles of GaN". Chemistry – A European Journal. 2 (11): 1353–1358. doi:10.1002/chem.19960021104.
- Wöhler, Lothar; Martin, F (1917). "Die Sensibilität der Azide". Angewandte Chemie. 30 (9): 33–39. Bibcode:1917AngCh..30...33W. doi:10.1002/ange.19170300902.
- ^ Li, Qian Shu; Duan, Hong Xia (2005-10-01). "Density Functional Theoretical Study of a Series of Binary Azides M(N 3 ) n ( n = 3, 4)". The Journal of Physical Chemistry A. 109 (40): 9089–9094. Bibcode:2005JPCA..109.9089L. doi:10.1021/jp052726t. ISSN 1089-5639. PMID 16332016.
- Hassner, Alfred; Stern, Meir; Gottlieb, Hugo E.; Frolow, Felix (1990). "Synthetic methods. 33. Utility of a polymeric azide reagent in the formation of di- and triazidomethane. Their NMR spectra and the x-ray structure of derived triazoles". The Journal of Organic Chemistry. 55 (8): 2304–2306. doi:10.1021/jo00295a014. ISSN 0022-3263.
- Banert, Klaus; Joo, Young-Hyuk; Rüffer, Tobias; Walfort, Bernhard; Lang, Heinrich (2007). "The Exciting Chemistry of Tetraazidomethane". Angewandte Chemie International Edition. 46 (7): 1168–1171. doi:10.1002/anie.200603960. PMID 17143916.
- Wiberg, Egon; Michaud, Horst (1954-07-01). "Notizen: Zur Kenntnis eines Siliciumtetrazids Si(N3)4". Zeitschrift für Naturforschung B. 9 (7): 500. doi:10.1515/znb-1954-0717. ISSN 1865-7117. S2CID 98275613.
- Filippou, Alexander C.; Portius, Peter; Schnakenburg, Gregor (2003-01-14). "The Hexaazidosilicate(IV) Ion: Synthesis, Properties, and Molecular Structure". Journal of the American Chemical Society. 124 (42): 12396–12397. doi:10.1021/ja0273187. PMID 12381165.
- Drake, J. E.; Hemmings, R. T. (1973-01-15). "Preparation and Characterization of Pseudohalogeno(methyl)germanes and Related Compounds". Canadian Journal of Chemistry. 51 (2): 302–311. doi:10.1139/v73-043. ISSN 0008-4042.
- Filippou, Alexander C.; Portius, Peter; Neumann, Dirk U.; Wehrstedt, Klaus-Dieter (2000-12-01). <4333::aid-anie4333>3.0.co;2-s "The Hexaazidogermanate(IV) Ion: Syntheses, Structures, and Reactions". Angewandte Chemie. 39 (23): 4333–4336. Bibcode:2000AngCh..39.4333F. doi:10.1002/1521-3773(20001201)39:23<4333::aid-anie4333>3.0.co;2-s. ISSN 1433-7851. PMID 29711897.
- ^ Peerless, Benjamin; Keane, Theo; Meijer, Anthony J. H. M.; Portius, Peter (2015). "Homoleptic low-valent polyazides of group 14 elements". Chemical Communications. 51 (35): 7435–7438. doi:10.1039/c5cc00259a. ISSN 1359-7345. PMID 25773494. S2CID 32981384.
- Campbell, Rory; Davis, Martin F.; Fazakerley, Mathew; Portius, Peter (2015-11-13). "Taming Tin(IV) Polyazides". Chemistry - A European Journal. 21 (51): 18690–18698. doi:10.1002/chem.201503478. ISSN 0947-6539. PMID 26767331.
- Wiberg, Egon; Michaud, Horst (1954-07-01). "Notizen: Zur Kenntnis eines Natrium-hexazido-stannats Na2Sn(N3)6". Zeitschrift für Naturforschung B. 9 (7): 500–501. doi:10.1515/znb-1954-0718. ISSN 1865-7117. S2CID 94917667.
- Möller, Heinz (1949). "Versuche zur Darstellung von Blei(IV)-azid-Verbindungen". Zeitschrift für anorganische Chemie. 260 (4–5): 249–254. doi:10.1002/zaac.19492600406. ISSN 0372-7874.
- Fehlhammer, Wolf Peter; Beck, Wolfgang (2013). "Azide Chemistry - An Inorganic Perspective, Part 1 Metal Azides: Overview, General Trends and Recent Developments". Zeitschrift für anorganische und allgemeine Chemie. 639 (7): 1053–1082. doi:10.1002/zaac.201300162.
- ^ Buder, Wolfgang; Schmidt, Armin (1975). "Phosphorazide und deren Schwingungsspektren". Chemischer Informationsdienst. 415 (3): 263–267. doi:10.1002/chin.197534036.
- Schulz, Axel; Villinger, Alexander (2012-03-05). "Binary Pnictogen Azides-An Experimental and Theoretical Study: [As(N3)4] , [Sb(N3)4], and [Bi(N3)5 (dmso)]". Chemistry - A European Journal. 18 (10): 2902–2911. doi:10.1002/chem.201102656. PMID 22337137.
- Schmidt, Armin (1970). "Darstellung und IR-Spektren der Dimethyldiazido-, Methyltriazido- und Tetraazidophosphonium-hexachloroantimonate(V)". Chemische Berichte. 103 (12): 3923–3927. doi:10.1002/cber.19701031223. ISSN 0009-2940.
- ^ Haiges, Ralf; Vij, Ashwani; Boatz, Jerry A.; Schneider, Stefan; Schroer, Thorsten; Gerken, Michael; Christe, Karl O. (2004-01-23). "First Structural Characterization of Binary AsIII and SbIII Azides". Chemistry - A European Journal. 10 (2): 508–517. doi:10.1002/chem.200305482. ISSN 0947-6539. PMID 14735519.
- ^ Geissler, Petra; Klapötke, Thomas M.; Kroth, Heinz-Jürgen (1995-06-01). "14N NMR spectra of covalent halogen and arsenic azides: XN3 (X Cl, Br, I), (CH3)nAs(N3)3−n (n = 0, 1, 2) and [As(N3)4]+". Spectrochimica Acta Part A: Molecular and Biomolecular Spectroscopy. 51 (6): 1075–1078. doi:10.1016/0584-8539(94)01313-6. ISSN 1386-1425.
- ^ Haiges, Ralf; Boatz, Jerry A.; Vij, Ashwani; Vij, Vandana; Gerken, Michael; Schneider, Stefan; Schroer, Thorsten; Yousufuddin, Muhammed; Christe, Karl O. (2004-12-10). "Polyazide Chemistry: Preparation and Characterization of As(N3)5, Sb(N3)5, and [P(C6H5)4][Sb(N3)6]". Angewandte Chemie International Edition. 43 (48): 6676–6680. doi:10.1002/anie.200461730. ISSN 1433-7851. PMID 15593158.
- ^ Karaghiosoff, Konstantin; Klapötke, Thomas M.; Krumm, Burkhard; Nöth, Heinrich; Schütt, Thomas; Suter, Max (2002-01-01). "Experimental and Theoretical Characterization of Cationic, Neutral, and Anionic Binary Arsenic and Antimony Azide Species". Inorganic Chemistry. 41 (2): 170–179. doi:10.1021/ic010463l. ISSN 0020-1669. PMID 11800605.
- ^ Villinger, Alexander; Schulz, Axel (2010-10-18). "Binary Bismuth(III) Azides: Bi(N3)3 , [Bi(N3)4]−, and [Bi(N3)6]3−". Angewandte Chemie International Edition. 49 (43): 8017–8020. doi:10.1002/anie.201002179. PMID 20839195.
- ^ Haiges, Ralf; Rahm, Martin; Dixon, David A.; Garner, Edward B.; Christe, Karl O. (2012-01-16). "Binary Group 15 Polyazides. Structural Characterization of [Bi(N 3 ) 4 ] − , [Bi(N 3 ) 5 ] 2– , [bipy·Bi(N 3 ) 5 ] 2– , [Bi(N 3 ) 6 ] 3– , bipy·As(N 3 ) 3 , bipy·Sb(N 3 ) 3 , and [(bipy) 2 ·Bi(N 3 ) 3 ] 2 and on the Lone Pair Activation of Valence Electrons". Inorganic Chemistry. 51 (2): 1127–1141. doi:10.1021/ic202307a. ISSN 0020-1669. PMID 22221015.
- Crawford, Margaret-Jane; Klapötke, Thomas M. (1999-06-01). "Possible Intermediate Formation of Cyclic Nitrous Oxide, N 2 O: Reaction of Oxygen Difluoride, OF2 , with Cesium Azide, CsN3". Inorganic Chemistry. 38 (12): 3006–3009. doi:10.1021/ic990240j. ISSN 0020-1669. PMID 11671052.
- Wang, Li Jie; Zgierski, Marek Z.; Mezey, Paul G. (2003-03-01). "Stable Structures of Nitrogen-Rich Sulfides: S(N 3 ) m ( m = 1−4)". The Journal of Physical Chemistry A. 107 (12): 2080–2084. Bibcode:2003JPCA..107.2080W. doi:10.1021/jp022074y. ISSN 1089-5639.
- Zeng, Xiaoqing; Beckers, Helmut; Bernhardt, Eduard; Willner, Helge (2011-09-05). "Synthesis and Characterization of Sulfuryl Diazide, O 2 S(N 3 ) 2". Inorganic Chemistry. 50 (17): 8679–8684. doi:10.1021/ic201294b. ISSN 0020-1669. PMID 21815651.
- Klapötke, Thomas M.; Krumm, Burkhard; Scherr, Matthias; Haiges, Ralf; Christe, Karl O. (2007-11-19). "The Binary Selenium(IV) Azides Se(N 3 ) 4 , [Se(N 3 ) 5 ] − , and [Se(N 3 ) 6 ] 2−". Angewandte Chemie International Edition. 46 (45): 8686–8690. doi:10.1002/anie.200702758. PMID 17935101.
- Johnson, James P.; MacLean, Gregory K.; Passmore, Jack; White, Peter S. (1989-11-01). "The preparation and X-ray crystal structure of Te(N 3 ) 3 SbF 6 containing the triazidotellurium(IV) cation, Te(N 3 ) 3 +". Canadian Journal of Chemistry. 67 (11): 1687–1692. doi:10.1139/v89-259. ISSN 0008-4042.
- Haiges, Ralf; Boatz, Jerry A.; Vij, Ashwani; Gerken, Michael; Schneider, Stefan; Schroer, Thorsten; Christe, Karl O. (2003-12-08). "Polyazide Chemistry: Preparation and Characterization of Te(N3)4 and[P(C6H5)4]2[Te(N3)6] and Evidence for[N(CH3)4][Te(N3)5]". Angewandte Chemie International Edition. 42 (47): 5847–5851. doi:10.1002/anie.200352680. ISSN 1433-7851. PMID 14673915.
- Bauer, S. H. (1947). "An Electron Diffraction Investigation of the Structure of Difluorodiazine". Journal of the American Chemical Society. 69 (12): 3104–3108. doi:10.1021/ja01204a053. ISSN 0002-7863.
- Milligan, Dolphus E.; Jacox, Marilyn E. (1964). "Infrared Spectra of NF, NCl, and NBr". The Journal of Chemical Physics. 40 (9): 2461–2466. Bibcode:1964JChPh..40.2461M. doi:10.1063/1.1725547. ISSN 0021-9606.
- ^ Dehnicke, K. (1967). "Reactions of Halogen Azides". Angewandte Chemie International Edition in English. 6 (3): 240–246. doi:10.1002/anie.196702401. ISSN 0570-0833.
- Dehnicke, Kurt (1979). "The Chemistry of Iodine Azide". Angewandte Chemie International Edition in English. 18 (7): 507–514. doi:10.1002/anie.197905071. ISSN 0570-0833.
- Hassner, Alfred; Marinescu, Lavinia; Bols, Mikael (2005-10-15), "Iodine Azide", in John Wiley & Sons, Ltd. (ed.), Encyclopedia of Reagents for Organic Synthesis, Chichester: John Wiley & Sons, Ltd, pp. ri007, doi:10.1002/047084289x.ri007, ISBN 978-0-471-93623-7, retrieved 2022-12-13
- Lyhs, Benjamin; Bläser, Dieter; Wölper, Christoph; Schulz, Stephan; Jansen, Georg (2012-12-14). "A Comparison of the Solid-State Structures of Halogen Azides XN 3 (X=Cl, Br, I)". Angewandte Chemie International Edition. 51 (51): 12859–12863. doi:10.1002/anie.201206028. PMID 23143850.