Revision as of 23:19, 1 November 2015 editEpipelagic (talk | contribs)Autopatrolled, Extended confirmed users, Pending changes reviewers85,809 edits →top: per talk← Previous edit | Revision as of 00:14, 4 November 2015 edit undoDrChrissy (talk | contribs)Extended confirmed users21,946 edits →Nervous system: deleted as per TalkNext edit → | ||
Line 143: | Line 143: | ||
===Nervous system=== | ===Nervous system=== | ||
In 2015, Lynne Sneddon, Director of Veterinary Science at the University of Liverpool, wrote "The neurophysiological basis of nociception or pain in fish is demonstrably similar to that in mammals."<ref name="Sneddon2015" /> | |||
====Receptors==== | ====Receptors==== |
Revision as of 00:14, 4 November 2015
![]() | The neutrality of this article is disputed. Relevant discussion may be found on the talk page. Please do not remove this message until conditions to do so are met. (October 2015) (Learn how and when to remove this message) |

Pain in fish is a contentious issue. Pain is a complex mental state, with a distinct perceptual quality but also associated with suffering, which is an emotional state. Because of this complexity, the presence of pain in an animal, or another human for that matter, cannot be determined unambiguously using observational methods, but the conclusion that animals experience pain is often inferred on the basis of likely presence of phenomenal consciousness which is deduced from comparative brain physiology as well as physical and behavioural reactions.
Fish fulfill several criteria proposed as indicating that non-human animals may experience pain. These fulfilled criteria include a suitable nervous system and sensory receptors, opioid receptors and reduced responses to noxious stimuli when given analgesics and local anaesthetics, physiological changes to noxious stimuli, displaying protective motor reactions, exhibiting avoidance learning and making trade-offs between noxious stimulus avoidance and other motivational requirements.
If fish feel pain, there are ethical and animal welfare implications including the consequences of exposure to pollutants, and practices involving commercial and recreational fishing, aquaculture, in ornamental fish and genetically modified fish and for fish used in scientific research.
Background
The possibility that fish and other non-human animals may experience pain has a long history. Initially, this was based around theoretical and philosophical argument, but more recently has turned to scientific investigation.
Philosophy

The idea that non-human animals might not feel pain goes back to the 17th-century French philosopher, René Descartes, who argued that animals do not experience pain and suffering because they lack consciousness. In 1789, the British philosopher and social reformist, Jeremy Bentham, addressed in his book An Introduction to the Principles of Morals and Legislation the issue of our treatment of animals with the following often quoted words: "The question is not, Can they reason? nor, can they talk? but, Can they suffer?"
Peter Singer, a bioethicist and author of Animal Liberation published in 1975, suggested that consciousness is not necessarily the key issue: just because animals have smaller brains, or are ‘less conscious’ than humans, does not mean that they are not capable of feeling pain. He goes on further to argue that we do not assume newborn infants, people suffering from neurodegenerative brain diseases or people with learning disabilities experience less pain than we would.
Bernard Rollin, the principal author of two U.S. federal laws regulating pain relief for animals, writes that researchers remained unsure into the 1980s as to whether animals experience pain, and veterinarians trained in the U.S. before 1989 were taught to simply ignore animal pain. In his interactions with scientists and other veterinarians, Rollin was regularly asked to "prove" that animals are conscious, and to provide "scientifically acceptable" grounds for claiming that they feel pain.
Continuing into the 1990's, discussions were further developed on the roles that philosophy and science had in understanding animal cognition and mentality. In subsequent years, it was argued there was strong support for the suggestion that some animals (most likely amniotes) have at least simple conscious thoughts and feelings and that the view animals feel pain differently to humans is now a minority view.
Scientific investigation
Cambridge Declaration on Consciousness (2012)The absence of a neocortex does not appear to preclude an organism from experiencing affective states. Convergent evidence indicates that non-human animals have the neuroanatomical, neurochemical, and neurophysiological substrates of conscious states along with the capacity to exhibit intentional behaviors. Consequently, the weight of evidence indicates that humans are not unique in possessing the neurological substrates that generate consciousness. Non-human animals, including all mammals and birds, and many other creatures, including octopuses, also possess these neurological substrates.
In the 20th- and 21st-century, there were many scientific investigations of pain in non-human animals.
Mammals
At the turn of the century, studies were published showing that arthritic rats self-select analgesic opiates. In 2014, the veterinary Journal of Small Animal Practice published an article on the recognition of pain which started - "The ability to experience pain is universally shared by all mammals..." and in 2015, it was reported in the science journal Pain, that several mammalian species (rat, mouse, rabbit, cat and horse) adopt a facial expression in response to a noxious stimulus that is consistent with the expression of humans in pain.
Birds
At the same time as the investigations using arthritic rats, studies were published showing that birds with gait abnormalities self-select for a diet that contains carprofen, a human analgesic. In 2005, it was written "Avian pain is likely analogous to pain experienced by most mammals" and in 2014, "...it is accepted that birds perceive and respond to noxious stimuli and that birds feel pain"
Reptiles and amphibians
Veterinary articles have been published stating both reptiles and amphibians experience pain in a way analogous to humans, and that analgesics are effective in these two classes of vertebrates.
Argument by analogy
In 2012 the American philosopher Gary Varner reviewed the research literature on pain in animals. His findings are summarised in the following table.
Argument by analogy | |||||||||
---|---|---|---|---|---|---|---|---|---|
Property | |||||||||
Fish | Amphibians | Reptiles | Birds | Mammals | |||||
Has nociceptors | ![]() |
![]() |
![]() |
![]() |
![]() | ||||
Has brain | ![]() |
![]() |
![]() |
![]() |
![]() | ||||
Nociceptors and brain linked | ![]() |
? / ![]() |
? / ![]() |
? / ![]() |
![]() | ||||
Has endogenous opioids | ![]() |
![]() |
![]() |
![]() |
![]() | ||||
Analgesics affect responses | ![]() |
? | ? | ![]() |
![]() | ||||
Response to damaging stimuli similar to humans | ![]() |
![]() |
![]() |
![]() |
![]() |
Notes
- But see
- But see
- But see
- But see
Arguing by analogy, Varner claims that any animal which exhibits the properties listed in the table could be said to experience pain. On that basis, he concludes that all vertebrates, including fish, probably experience pain, but invertebrates apart from cephalopods probably do not experience pain.
The experience of pain
Although there are numerous definitions of pain, almost all involve two key components.
First, nociception is required. This is the ability to detect noxious stimuli which evoke a reflex response that rapidly moves the entire animal, or the affected part of its body, away from the source of the stimulus. The concept of nociception does not imply any adverse, subjective "feeling" – it is a reflex action. An example in humans would be the rapid withdrawal of a finger that has touched something hot – the withdrawal occurs before any sensation of pain is actually experienced.
The second component is the experience of "pain" itself, or suffering – the internal, emotional interpretation of the nociceptive experience. Again in humans, this is when the withdrawn finger begins to hurt, moments after the withdrawal. Pain is therefore a private, emotional experience. Pain cannot be directly measured in other animals, including other humans; responses to putatively painful stimuli can be measured, but not the experience itself. To address this problem when assessing the capacity of other species to experience pain, argument-by-analogy is used. This is based on the principle that if an animal responds to a stimulus in a similar way to ourselves, it is likely to have had an analogous experience.
Nociception
Main article: Nociception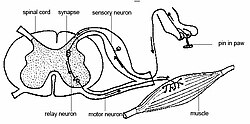
Nociception usually involves the transmission of a signal along a chain of nerve fibers from the site of a noxious stimulus at the periphery to the spinal cord and brain. This process evokes a reflex arc response generated at the spinal cord and not involving the brain, such as flinching or withdrawal of a limb. Nociception is found, in one form or another, across all major animal taxa. Nociception can be observed using modern imaging techniques; and a physiological and behavioral response to nociception can be detected.
Emotional pain
Main article: Psychological painSometimes a distinction is made between "physical pain" and "emotional" or "psychological pain". Emotional pain is the pain experienced in the absence of physical trauma, e.g. the pain experienced by humans after the loss of a loved one, or the break-up of a relationship. It has been argued that only primates and humans can feel "emotional pain", because they are the only animals that have a neocortex - a part of the brain's cortex considered to be the "thinking area". However, research has provided evidence that monkeys, dogs, cats and birds can show signs of emotional pain and display behaviours associated with depression during painful experience, i.e. lack of motivation, lethargy, anorexia, unresponsiveness to other animals.
Physical pain
Main article: PainThe nerve impulses of the nociception response may be conducted to the brain thereby registering the location, intensity, quality and unpleasantness of the stimulus. This subjective component of pain involves conscious awareness of both the sensation and the unpleasantness (the aversive, negative affect). The brain processes underlying conscious awareness of the unpleasantness (suffering), are not well understood.
There have been several published lists of criteria for establishing whether non-human animals experience pain, e.g. Some criteria that may indicate the potential of another species, including fishes, to feel pain include:
- Has a suitable nervous system and sensory receptors
- Has opioid receptors and shows reduced responses to noxious stimuli when given analgesics and local anaesthetics
- Physiological changes to noxious stimuli
- Displays protective motor reactions that might include reduced use of an affected area such as limping, rubbing, holding or autotomy
- Shows avoidance learning
- Shows trade-offs between noxious stimulus avoidance and other motivational requirements
- High cognitive ability and sentience
Research findings
Nervous system
Receptors

Primitive fish such as lampreys (Petromyzon marinus) have free nerve endings in the skin that respond to heat and mechanical pressure. However, behavioural reactions associated with nociception have not been recorded and it is also difficult to determine whether the mechanoreceptors in lamprey are truly nociceptive-specific or simply pressure-specific.
Early studies found polymodal nociceptors on the face and snout of rainbow trout (Oncorhynchus mykiss). These receptors respond to mechanical pressure, temperatures in the noxious range (> 40 °C) and 1% acetic acid (a chemical irritant). Stimulation of these causes an electrical signal to be sent to the brain via the trigeminal nerve. Further studies found nociceptors to be more widely distributed over the bodies of rainbow trout, and also those of cod and carp. The most sensitive areas of the body are around the eyes, nostrils, fleshy parts of the tail and the pectoral and dorsal fins.
Rainbow trout also have corneal nociceptors. Out of 27 receptors investigated in one study, seven were polymodal nociceptors and six were mechanothermal nociceptors. Mechanical and thermal thresholds were lower than those of cutaneous receptors, indicating greater sensitivity in the cornea.
It has been concluded that bony fish possess nociceptors that are similar in function to those in mammals.
Nerve fibres
There are two types of nerve fibre relevant to pain in fish. Group C nerve fibres are a type of sensory nerve fibre which lack a myelin sheath and have a small diameter, meaning they have a low nerve conduction velocity. The suffering that humans associate with burns, toothaches, or crushing injury are caused by C fibre activity. A typical human cutaneous nerve contains 83% Group C nerve fibres. A-delta fibres are another type of sensory nerve fibre, however, these are myelinated and therefore transmit impulses faster than non-myelinated C fibres. A-delta fibres carry cold, pressure and some pain signals, and are associated with acute pain that results in "pulling away" from noxious stimuli.
In bony fish, Group C and A-delta fibres (combined) represent 38.7% of the fibres in the tail nerves of common carp and 36% of the trigeminal nerve of rainbow trout. However, only 5% of these are C fibres in the carp, slightly more than the 4% C fibres reported in the rainbow trout.
Some species of cartilagenous fish possess A-delta fibres, however, C fibres are either absent or found in very low numbers.
Brain

Somatosensory evoked potentials (SEPs) are weak electric responses in the central nervous system (CNS) following stimulation of peripheral sensory nerves. These indicate there is a pathway from nociceptors in the periphery to higher brain regions. In goldfish (Carassius auratus), rainbow trout, Atlantic salmon (Salmo salar) and Atlantic cod (Gadus morhua), it has been demonstrated that putatively non-noxious and noxious stimulation elicit SEPs in different brain regions, including the telencephalon which may mediate the co-ordination of pain information.
If sensory responses in fish are limited to the spinal cord and hindbrain, they might be considered as simply reflexive. However, microarray analysis of gene expression shows the brain is active at the molecular level in the forebrain, midbrain and hindbrain of common carp and rainbow trout. Several genes that are involved in mammalian nociception, such as brain-derived neurotrophic factor (BDNF) and the cannabinoid CB1 receptor were regulated in the fish brain after a nociceptive event.
It has been concluded that the brains of rainbow trout fire neurons in the same way human brains do when experiencing pain.
Opioid system and effects of analgesics

Teleost fish have a functional opioid system which includes the presence of opioid receptors similar to those of mammals. Veterinary medicine uses for fish, the same analgesics and anesthetics used in humans and other mammals. These chemicals act on the nociceptive pathways, blocking signals to the brain where emotional responses to the signals are further processed by certain parts of the brain found in amniotes ("higher vertebrates").
Effects of morphine

Pre-treatment with morphine (an analgesic in humans and other mammals) has a dose-dependent anti-nociceptive effect and mitigates the behavioural and ventilation rate responses of rainbow trout to noxious stimuli.
When acetic acid is injected into the lips of rainbow trout, they exhibit anomalous behaviours such as side-to-side rocking and rubbing their lips along the sides and floors of the tanks, and their ventilation rate increases. Injections of morphine reduce both the anomalous, noxious-stimulus related behaviours and the increase in ventilation rate. When the same noxious stimulus is applied to zebrafish (Danio rerio), they respond by decreasing their activity. As with the rainbow trout, morphine injected prior to the acid injection attenuates the decrease in activity in a dose-dependent manner.
Injection of acetic acid into the lips of rainbow trout causes a reduction in their natural neophobia (fear of novelty); this is reversed by the administration of morphine.
In goldfish injected with morphine or saline and then exposed to to unpleasant temperatures, fish injected with saline acted with defensive behaviours indicating anxiety, wariness and fear, whereas those given morphine did not.
Effects of other analgesics
The neurotransmitter, Substance P and the analgesic opioid enkephalins and β-endorphin, which act as endogenous analgesics in mammals, are present in fish.
Different analgesics have different effects on fish. In a study on the efficacy of three types of analgesic, buprenorphine (an opioid), carprofen (a non-steroidal anti-inflammatory drug) and lidocaine (a local anaesthetic), ventilation rate and time to resume feeding were used as pain indicators. Buprenorphine had limited impact on the fish's response, carprofen ameliorated the effects of noxious stimulation on time to resume feeding, however, lidocaine reduced all the behavioural indicators.
Tramadol also increases the nociceptive threshold in fish, providing further evidence of an anti-nociceptive opioid system in fish.
Effects of naloxone
Naloxone is an μ-opioid receptor antagonist which, in mammals, negates the analgesic effects of opioids. Both adult and 5-day old zebrafish larvae show behavioural responses indicative of pain in response to injected or diluted acetic acid. The anti-nociceptive properties of morphine or buprenorphine are reversed if adults, or larvae, are co-treated with naloxone. Both naloxone and prolyl-leucyl-glycinamide (another opiate antagonist in mammals) reduced the analgesic effects of morphine to electric shocks received by goldfish, indicating they can act as an opiate antagonist in fish.
Physiological changes
The physiological changes of fish in response to noxious stimuli include elevations of ventilation rate and cortisol levels.
Protective responses



Studies show that fish exhibit protective behavioural responses to putatively painful stimuli.
When acetic acid or bee venom is injected into the lips of rainbow trout, they exhibit an anomalous side-to-side rocking behaviour on their pectoral fins, rub their lips along the sides and floors of the tanks and increase their ventilation rate. When acetic acid is injected into the lips of zebrafish, they respond by decreasing their activity. The magnitude of this behavioural response depends on the concentration of the acetic acid.
The behavioural responses to a noxious stimulus differ between species of fish. Noxiously stimulated common carp (Cyprinus carpio) show anomalous rocking behaviour and rub their lips against the tank walls, but do not change other behaviours or their ventilation rate. In contrast, zebrafish (Danio rerio) reduce their frequency of swimming and increase their ventilation rate but do not display anomalous behaviour. Rainbow trout, like the zebrafish, reduce their frequency of swimming and increase their ventilation rate. Nile tilapia (Oreochromis niloticus), in response to a tail fin clip, increase their swimming activity and spend more time in the light area of their tank.
Since this initial work, Lynne Sneddon and her co-workers have characterised pain responses in rainbow trout, common carp and zebrafish.
Five-day old zebrafish larvae show a concentration dependent increase in locomotor activity in response to different concentrations of diluted acetic acid. This increase in locomotor activity is accompanied by an increase in cox-2 mRNA, demonstrating that nociceptive pathways are also activated.
Fish show different responses to different noxious stimuli, even when these are apparently similar. This indicates the response is flexible and not simply a nociceptive reflex. Atlantic cod injected in the lip with acetic acid, capsaicin, or piercing the lip with a commercial fishing hook, showed different responses to these three types of noxious stimulation. Those cod treated with acetic acid and capsaicin displayed increased hovering close to the bottom of the tank and reduced use of shelter. However, hooked cod only showed brief episodes of head shaking.
Avoidance learning
Early experiments provided evidence that fish learn to respond to putatively noxious stimuli. For instance, toadfish (Batrachoididae) grunt when they are electrically shocked, but after repeated shocks, they grunt simply at the sight of the electrode. More recent studies show that both goldfish and trout learn to avoid locations in which they receive electric shocks. Furthermore, this avoidance learning is flexible and is related to the intensity of the stimulus.
Trade-offs in motivation
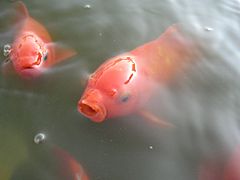
A painful experience may change the motivation for normal behavioural responses.
In a 2007 study, goldfish were trained to feed at a location of the aquarium where subsequently they would receive an electric shock. The number of feeding attempts and time spent in the feeding/shock zone decreased with increased shock intensity and with increased food deprivation the number and the duration of feeding attempts increased as did escape responses as this zone was entered. The researchers suggested that goldfish make a trade-off in their motivation to feed with their motivation to avoid an acute noxious stimulus.
Rainbow trout naturally avoid novelty (i.e. they are neophobic). Braithwaite describes a study in which a brightly coloured Lego brick is placed in the tank of rainbow trout. Trout injected in the lip with a small amount of saline strongly avoided the Lego brick, however, trout injected with acetic acid spent considerably more time near the Lego block. When the study was repeated but with the fish also being given morphine, the avoidance response returned in those fish injected with acetic acid and could not be distinguished from the responses of saline injected fish.
To explore the possibility of a trade-off between responding to a noxious stimulus and predation, researchers presented rainbow trout with a competing stimulus, a predator cue. Noxiously stimulated fish cease showing anti-predator responses, indicating that pain becomes their primary motivation. The same study investigated the potential trade-off between responding to a noxious stimulus and social status. The responses of the noxiously treated trout varied depending on the familiarity of the fish they were placed with. The researchers suggested the findings of the motivational changes and trade-offs provide evidence for central processing of pain rather than merely showing a nociceptive reflex.
Paying a cost for analgesia
Zebrafish given access to a barren, brightly lit chamber or an enriched chamber prefer the enriched area. When these fish are injected with acetic acid or saline as a control they still choose the same enriched chamber. However, if an analgesic is dissolved in the barren, less-preferred chamber, zebrafish injected with noxious acid lose their preference and spend over half their time in the previously less-favourable, barren chamber. This suggests a trade-off in motivation and furthermore, they are willing to pay a cost to enter a less preferred environment to access pain relief.
Cognitive ability and sentience
The learning abilities of fish demonstrated in a range of studies indicate sophisticated cognitive processes that are more complex than simple associative learning. Examples include the ability to recognise social companions, avoidance (for some months or years) of places where they encountered a predator or were caught on a hook and forming mental maps.
It has been argued that although a high cognitive capacity may indicate a greater likelihood of experiencing pain, it also gives these animals a greater ability to deal with this, leaving animals with a lower cognitive ability a greater problem in coping with pain.
Criteria for pain perception
Scientists have also proposed that in conjunction with argument-by-analogy, criteria of physiology or behavioural responses can be used to assess the possibility of non-human animals perceiving pain. The following is a table of criteria suggested by Sneddon et al.
Criteria for pain reception in fish | ||||
---|---|---|---|---|
Criteria | ||||
Jawless fish | Cartilaginous fish | Bony fish | Lobe-finned fish | |
Has nociceptors | ? | ? | ![]() |
? |
Pathways to central nervous system | ? | ? | ![]() |
? |
Central processing in brain | ? | ? | ![]() |
? |
Receptors for analgesic drugs | ? | ? | ![]() |
? |
Physiological responses | ? | ? | ![]() |
? |
Movement away from noxious stimuli | ? | ? | ![]() |
? |
Behavioural changes from norm | ? | ? | ![]() |
? |
Protective behaviour | ? | ? | ![]() |
? |
Responses reduced by analgesic drugs | ? | ? | ![]() |
? |
Self administration of analgesia | ? | ? | ? | ? |
Responses with high priority over other stimuli | ? | ? | ![]() |
? |
Pay cost to access analgesia | ? | ? | ? | ? |
Altered behavioural choices/preferences | ? | ? | ![]() |
? |
Relief learning | ? | ? | ? | ? |
Rubbing, limping or guarding | ? | ? | ![]() |
? |
Paying a cost to avoid stimulus | ? | ? | ? | ? |
Tradeoffs with other requirements | ? | ? | ![]() |
? |
In the table, Y indicates positive evidence and ? denotes it has not been tested or there is insufficient evidence.
Societal implications

Both scientists and animal protection advocates have raised concerns about the possible suffering (pain and fear) of fish caused by angling.
Other societal implications of fish experiencing pain include acute and chronic exposure to pollutants, commercial and sporting fisheries (e.g. injury during trawling, tagging/fin clipping during stock assessment, tissue damage, physical exhaustion and severe oxygen deficit during capture, pain and stress during slaughter, use of live bait), aquaculture (e.g. tagging/fin clipping, high stocking densities resulting in increased aggression, food deprivation for disease treatment or before harvest, removal from water for routine husbandry, pain during slaughter), ornamental fish (e.g. capture by sub-lethal poisoning, permanent adverse physical states due to selective breeding), scientific research (e.g. genetic-modification may have detrimental effects on welfare, deliberately-imposed adverse physical, physiological and behavioural states, electrofishing, tagging, fin clipping or otherwise marking fish, handling procedures which may cause injury.
Legislation
In the UK, the legislation protecting animals during scientific research, the "Animals (Scientific Procedures) Act 1986", protects fish from the moment they become capable of independent feeding. The legislation protecting animals in most other circumstances in the UK is "The Animal Welfare Act, 2006" which states that in the Act, " “animal” means a vertebrate other than man", clearly including fish.
In the US, the legislation protecting animals during scientific research is "The Animal Welfare Act". This excludes protection of "cold-blooded" animals, including fish.
The 1974 Norwegian Animal Rights Law states it relates to mammals, birds, frogs, salamander, reptiles, fish, and crustaceans.
Controversy
Nervous system
Receptors and nerve fibres
It has been argued that fish can not feel pain because they do not have a sufficient density of appropriate nerve fibres. A typical human cutaneous nerve contains 83% Group C nerve fibres, however, the same nerves in humans with congenital insensitivity to pain have only 24-28% C type fibres. Based on this, James Rose, from the University of Wyoming, has argued that the absence of C type fibres in cartilagenous sharks and rays indicates that signalling leading to pain perception is likely to be impossible, and the low numbers for bony fish (e.g. 5% for carp and trout) indicate this is also highly unlikely for these fish. Rose concludes there is little evidence that sharks and rays possess the nociceptors required to initiate pain detection in the brain, and that, while bony fish are able to unconsciously learn to avoid injurious stimuli, they are little more likely to experience conscious pain than sharks.
A-delta type fibres, believed to trigger avoidance reactions, are common in bony fish, although they have not been found in sharks or rays.
Rose concludes that fishes have survived well in an evolutionary sense without the full range of nociception typical of humans or other mammals.
Brain
In 2002, Rose published reviews arguing that fish cannot feel pain because they lack a neocortex in the brain. However, in 2003, a research team led by Lynne Sneddon concluded that the brains of rainbow trout fire neurons in the same way human brains do when experiencing pain. Rose criticized the study, claiming it was flawed, mainly because it did not provide proof that fish possess "conscious awareness, particularly a kind of awareness that is meaningfully like ours".
Rose, and more recently Brian Key from The University of Queensland, argue that because the fish brain is very different to humans, fish are probably not conscious in the manner humans are, and while fish may react in a way similar to the way humans react to pain, the reactions in the case of fish have other causes. Studies indicating that fish can feel pain were confusing nociception with feeling pain, says Rose. "Pain is predicated on awareness. The key issue is the distinction between nociception and pain. A person who is anaesthetised in an operating theatre will still respond physically to an external stimulus, but he or she will not feel pain." According to Rose and Key, the literature relating to the question of consciousness in fish is prone to anthropomorphisms and care is needed to avoid erroneously attributing human-like capabilities to fish. Sneddon suggests it is entirely possible that a species with a different evolutionary path could evolve different neural systems to perform the same functions, as studies on the brains of birds have shown. Key agrees that phenomenal consciousness is likely to occur in mammals and birds, but not in fish. Animal behaviouralist Temple Grandin argues that fish could still have consciousness without a neocortex because "different species can use different brain structures and systems to handle the same functions." Sneddon proposes that to suggest a function suddenly arises without a primitive form defies the laws of evolution. Other researchers also believe that animal consciousness does not require a neocortex, but can arise from homologous subcortical brain networks.
Protective responses
Initial work by Sneddon and her co-workers characterised behavioural responses in rainbow trout, common carp and zebrafish. However, when these experiments were repeated by Newby and Stevens without anaesthetic, rocking and rubbing behaviour was not observed, suggesting that some of the alleged pain responses observed by Sneddon and co-workers were likely to be due to recovery of the fish from anaesthesia.
Several researchers argue about the definition of pain used in behavioural studies, as the observations recorded were contradictory, non-validated and non-repeatable by other researchers. In 2012, Rose argued that fishes resume "normal feeding and activity immediately or soon after surgery".
Nordgreen said that the behavioural differences they found in response to uncomfortable temperatures showed that fish feel both reflexive and cognitive pain. "The experiment shows that fish do not only respond to painful stimuli with reflexes, but change their behavior also after the event," Nordgreen said. "Together with what we know from experiments carried out by other groups, this indicates that the fish consciously perceive the test situation as painful and switch to behaviors indicative of having been through an aversive experience." In 2012, Rose and others reviewed this and further studies which concluded that pain had been found in fish. They concluded that the results from such research are due to poor design and misinterpretation, and that the researchers were unable to distinguish unconscious detection of injurious stimuli (nociception) from conscious pain.
See also
- Animal cognition
- Animal consciousness
- Animal cruelty
- Eating live fish
- Ethics of eating meat
- Moral status of animals in the ancient world
- Pain and suffering in laboratory animals
- Sentience
References
- Abbott, F.V., Franklin, K.B.J. and Westbrook, R.F. (1995). "The formalin test: Scoring properties of the first and second phases of the pain response in rats". Pain. 60 (1): 91–102. doi:10.1016/0304-3959(94)00095-V. PMID 7715946.
{{cite journal}}
: CS1 maint: multiple names: authors list (link) - ^ Key, B. (2015). "Fish do not feel pain and its implications for understanding phenomenal consciousness". Biology and Philosophy. 30 (2): 149–165. doi:10.1007/s10539-014-9469-4.
- ^ Carbone, L. (2004). What Animals Want: Expertise and Advocacy in Laboratory Animal Welfare Policy. Oxford University Press. p. 149.
- Radner, D. and Radner, M. (1989). Animal Consciousness. Prometheus Books: Buffalo.
{{cite book}}
: CS1 maint: multiple names: authors list (link) - Harrison, P. (1992). "Descartes on animals". The Philosophical Quarterly. 42 (167): 219–227. doi:10.2307/2220217.
- "Bentham, J. (1879). An Introduction to the Principles of Morals and Legislation. Clarendon Press.
- ^ Sneddon, L.U. "Can animals feel pain?". The Welcome Trust. Retrieved September 24, 2015.
- ^ Rollin, B. (1989). The Unheeded Cry: Animal Consciousness, Animal Pain, and Science. Oxford University Press, pp. xii, 117-118, cited in Carbone 2004, p. 150.
- Allen, C. (1998). "Assessing animal cognition: Ethological and philosophical perspectives". Journal of Animal Science. 76 (1): 42–47. PMID 9464883.
- Griffin, D.R. and Speck, G.B. (2004). "New evidence of animal consciousness". Animal Cognition. 7 (1): 5–18. doi:10.1007/s10071-003-0203-x. PMID 14658059.
{{cite journal}}
: CS1 maint: multiple names: authors list (link) - ^ Low, P. (July 7, 2012). Jaak Panksepp, Diana Reiss, David Edelman, Bruno Van Swinderen, Philip Low and Christof Koch (ed.). "The Cambridge declaration on consciousness" (PDF). University of Cambridge.
{{cite web}}
: CS1 maint: multiple names: editors list (link) - Colpaert, F.C., Tarayre, J.P., Alliaga, M., Slot. L.A.B., Attal, N. and Koek, W. (2001). "Opiate self-administration as a measure of chronic nociceptive pain in arthritic rats". Pain. 91: 33–45. doi:10.1016/s0304-3959(00)00413-9.
{{cite journal}}
: CS1 maint: multiple names: authors list (link) - Mathews, K., Kronen, P.W., Lascelles, D., Nolan, A., Robertson, S., Steagall, P.V., Wright, B. and Yamashita, K. (2014). "Guidelines for recognition, assessment and treatment of pain". Journal of Small Animal Practice. 55 (6): E10–E68.
{{cite journal}}
: CS1 maint: multiple names: authors list (link) - Chambers, C.T. and Mogil,J.S. (2015). "Ontogeny and phylogeny of facial expression of pain". Pain. 156 (5): 798–799. doi:10.1097/j.pain.0000000000000133.
{{cite journal}}
: CS1 maint: multiple names: authors list (link) - Danbury, T.C., Weeks, C.A., Chambers, J.P., Waterman-Pearson, A.E. and Kestin, S.C. (2000). "Self-selection of the analgesic drug carprofen by lame broiler chickens". The Veterinary Record. 146 (11): 307–311.
{{cite journal}}
: CS1 maint: multiple names: authors list (link) - Machin, K.L. (2005). "Avian analgesia". Seminars in Avian and Exotic Pet Medicine. 14 (4): 236–242. doi:10.1053/j.saep.2005.09.004.
- Paul-Murphy, J. and Hawkins, M.G. (2014). "Chapter 26 - Bird-specific considerations: recognizing pain in pet birds.". In Gaynor, J.S. and Muir III, W. W. (ed.). Handbook of Veterinary Pain Management. Elsevier Health Sciences.
{{cite book}}
: CS1 maint: multiple names: authors list (link) - Mosley, C.A. (2005). "Anesthesia and analgesia in reptiles". Seminars in Avian and Exotic Pet Medicine. 14 (4): 243–262. doi:10.1053/j.saep.2005.09.005.
- Mosley, C. (2011). "Pain and nociception in reptiles". Veterinary Clinics of North America: Exotic Animal Practice. 14 (1): 45–60. doi:10.1016/j.cvex.2010.09.009.
- Sladky, K.K. and Mans, C. (2012). "Clinical analgesia in reptiles". Journal of Exotic Pet Medicine. 21 (2): 158–167. doi:10.1053/j.jepm.2012.02.012.
{{cite journal}}
: CS1 maint: multiple names: authors list (link) - Machin, K.L. (1999). "Amphibian pain and analgesia". Journal of Zoo and Wildlife Medicine: 2–10.
- Machin, K.L. (2001). "Fish, amphibian, and reptile analgesia". The Veterinary Clinics of North America. Exotic Animal Practice. 4 (1): 19–33.
- Stevens, C.W. (2011). "Analgesia in amphibians: preclinical studies and clinical applications". Veterinary Clinics of North America: Exotic Animal Practice. 14 (1): 33–44. doi:10.1016/j.cvex.2010.09.007.
- ^ Varner, Gary E. (2012) "Which Animals Are Sentient?" Chapter 5 in: Personhood, Ethics, and Animal Cognition: Situating Animals in Hare’s Two Level Utilitarianism, Oxford University Press. ISBN 9780199758784. doi:10.1093/acprof:oso/9780199758784.001.0001 The table in the article is based on table 5.2, page 113.
- Guénette, S.A., Giroux, M.C. and Vachon, P. (2013). "Pain perception and anaesthesia in research frogs". Experimental Animals. 62 (2): 87–92.
{{cite journal}}
: CS1 maint: multiple names: authors list (link) - Mosley, C. (2006). "Pain, nociception and analgesia in reptiles: when your snake goes 'ouch!'" (PDF). The North American Veterinary Conference. 20: 1652–1653.
- Coble, D.J., Taylor, D.K. and Mook, D.M. (2011). "Analgesic effects of meloxicam, morphine sulfate, flunixin meglumine, and xylazine hydrochloride in African-clawed frogs (Xenopus laevis)". Journal of the American Association for Laboratory Animal Science. 50 (3): 355.
{{cite journal}}
: CS1 maint: multiple names: authors list (link) - Baker, B.B., Sladky, K.K. and Johnson, S.M. (2011). "Evaluation of the analgesic effects of oral and subcutaneous tramadol administration in red-eared slider turtles". Journal of the American Veterinary Medical Association. 238 (2): 220–227. doi:10.2460/javma.238.2.220.
{{cite journal}}
: CS1 maint: multiple names: authors list (link) - Andrews, Kristin (2014) The Animal Mind: An Introduction to the Philosophy of Animal Cognition section 3.6.2, Routledge. ISBN 9781317676751.
- ^ Sneddon, L.U. (2004). "Evolution of nociception in vertebrates: comparative analysis of lower vertebrates". Brain Research Reviews. 46: 123–130. doi:10.1016/j.brainresrev.2004.07.007.
- ^ Sneddon, L.U., Elwood, R.W., Adamo, S.A. and Leach, M.C. (2014). "Defining and assessing animal pain". Animal Behaviour. 97: 201-212.
{{cite journal}}
: CS1 maint: multiple names: authors list (link) - ^ Elwood, R.W., Barr, S. and Patterson, L. (2009). "Pain and stress in crustaceans?". Applied Animal Behaviour Science. 118 (3): 128–136.
{{cite journal}}
: CS1 maint: multiple names: authors list (link) - ^ Huntingford, F.A., Adams, C., Braithwaite, V.A., Kadri, S., Pottinger, T.G., Sandøe, P. and Turnbull, J.F. (2006). "Review paper: Current issues in fish welfare" (PDF). Journal of Fish Biology. 68 (2): 332–372. doi:10.1111/j.0022-1112.2006.001046.x.
{{cite journal}}
: CS1 maint: multiple names: authors list (link) - ^ Sneddon, L.U., Braithwaite, V.A. and Gentle, M.J. (2003). "Do fish have nociceptors: Evidence for the evolution of a vertebrate sensory system". Proceedings of the Royal Society: Biological Sciences. 270 (1520): 1115–1121. doi:10.1098/rspb.2003.2349.
{{cite journal}}
: CS1 maint: multiple names: authors list (link) - Sneddon L.U. (2003). "Trigeminal somatosensory innervation of the head of the rainbow trout with particular reference to nociception". Brain Research. 972: 44–52.
- Ashley, P.J., Sneddon L.U. and McCrohan C.R. (2007). "Nociception in fish: stimulus–response properties of receptors on the head of trout Oncorhynchus mykiss". Brain Research. 1166: 47–54.
{{cite journal}}
: CS1 maint: multiple names: authors list (link) - Mettam J.J., McCrohan C.R. and Sneddon L.U. (2011). "Characterisation of chemosensory trigeminal receptors in the rainbow trout (Oncorhynchus mykiss): responses to irritants and carbon dioxide". Journal of Experimental Biology. 215: 685–693.
- ^ Braithwaite, V. (2010). Do Fish Feel Pain?. Oxford University Press.
- Ashley, P.J., Sneddon, L.U. and McCrohan, C.R. (2006). "Properties of corneal receptors in a teleost fish". Neuroscience Letters. 410: 165–168.
{{cite journal}}
: CS1 maint: multiple names: authors list (link) - Sneddon, L.U. (2015). "Pain in aquatic animals". The Journal of Experimental Biology. 218 (7): 967–976. doi:10.1242/jeb.088823.
- ^ Correia, A.D., Cunha, S.R., Scholze, M. and Stevens, E.D. (2011). "A novel behavioral fish model of nociception for testing analgesics". Pharmaceuticals. 4 (4): 665–680.
{{cite journal}}
: CS1 maint: multiple names: authors list (link) - ^ Rose, J.D., Arlinghaus, R., Cooke, S.J., Diggles, B.K., Sawynok, W., Stevens, E.D. and Wynne, C.D.L. (2012). "Can fish really feel pain?" (PDF). Fish and Fisheries. 15 (1): 97–133. doi:10.1111/faf.12010.
{{cite journal}}
: CS1 maint: multiple names: authors list (link) - Sneddon, L.U. (2002). "Anatomical and electrophysiological analysis of the trigeminal nerve of the rainbow trout, Onchorynchus mykiss". Neuroscience Letters. 319 (3): 167–171. doi:10.1016/S0304-3940(01)02584-8.
- Snow, P.J., Plenderleith, M.B. and Wright L.L. (1993). "Quantitative study of primary sensory neurone populations of three species of elasmobranch fish". Journal of Comparative Neurology. 334: 97–103.
{{cite journal}}
: CS1 maint: multiple names: authors list (link) - Braithwaite, V.A. and Boulcott, P. (2007). "Pain perception, aversion and fear in fish" (PDF). Diseases of Aquatic Organisms. 75 (2): 131–138.
{{cite journal}}
: CS1 maint: multiple names: authors list (link) - Ludvigsen, S., Stenklev, N.C., Johnsen, H.K., Laukli, E., Matre, D. and Aas-Hansen, Ø. (2014). "Evoked potentials in the Atlantic cod following putatively innocuous and putatively noxious electrical stimulation: a minimally invasive approach". Fish Physiology and Biochemistry. 40 (1): 173–181. doi:10.1007/s10695-013-9834-2.
{{cite journal}}
: CS1 maint: multiple names: authors list (link) - ^ Reilly, S.C., Quinn, J.P., Cossins, A.R. and Sneddon L.U. (2008). "Novel candidate genes identified in the brain during nociception in common carp (Cyprinus carpio) and rainbow trout (Oncorhynchus mykiss)". Neuroscience Letters. 437 (2): 135–138. doi:10.1016/j.neulet.2008.03.075.
{{cite journal}}
: CS1 maint: multiple names: authors list (link) - ^ "Fish do feel pain, scientists say". BBC News. 30 April 2003. Retrieved 20 May 2010.
- ^ Grandin, T. and Johnson, C. (2005). Animals in Translation. New York: Scribner. pp. 183–184. ISBN 0-7432-4769-8.
{{cite book}}
: CS1 maint: multiple names: authors list (link) - Buatti, M.C. and Pasternak, G.W. (1981). "Multiple opiate receptors: phylogenetic differences". Brain Research. 218: 400–405. doi:10.1016/0006-8993(81)91319-6.
{{cite journal}}
: CS1 maint: multiple names: authors list (link) - Velasco, E.M.F., Law, P.Y. and Rodriguez, R.E. (2009). "Mu opioid receptor from the zebrafish exhibits functional characteristics as those of mammalian Mu opioid receptor". Zebrafish. 6: 259–268. doi:10.1089/zeb.2009.0594.
{{cite journal}}
: CS1 maint: multiple names: authors list (link) - Viñuela-Fernández I, Jones E, Welsh EM, Fleetwood-Walker SM (September 2007). "Pain mechanisms and their implication for the management of pain in farm and companion animals". Vet. J. 174 (2): 227–39. doi:10.1016/j.tvjl.2007.02.002. PMID 17553712.
{{cite journal}}
: CS1 maint: multiple names: authors list (link) - Sneddon, L.U. (2012). "Clinical anesthesia and analgesia in fish". Journal of Exotic Pet Medicine. 21: 32–43.
- ^ Jones, S.G., Kamunde, C., Lemke, K. and Stevens, E.D. (2012). "The dose-response relation for the antinociceptive effect of morphine in a fish, rainbow trout". Journal of Veterinary Pharmacological Therapy. 35: 563–570. doi:10.1111/j.1365-2885.2011.01363.x.
{{cite journal}}
: CS1 maint: multiple names: authors list (link) - ^ Sneddon, L.U. (2003). "The evidence for pain in fish: The use of morphine as an analgesic". Applied Animal Behaviour Science. 83 (2): 153–162.
- Nordgreen, J., Joseph, P., Garner, J.P., Janczak, A.M., Ranheim, B., Muir, W.M. and Horsberg, T.E. (2009). "Thermonociception in fish: Effects of two different doses of morphine on thermal threshold and post-test behaviour in goldfish (Carassius auratus)". Applied Animal Behaviour Science. 119 (1–2): 101–107.
{{cite journal}}
: CS1 maint: multiple names: authors list (link) - ^ Broom, D.M. (2007). "Cognitive ability and sentience: Which aquatic animals should be protected?" (PDF). Diseases of Aquatic Organisms. 75 (2): 99–108.
- Mettam, J.J., Oulton, L.J., McCrohan, C.R. and Sneddon, L.U. (2011). "The efficacy of three types of analgesic drugs in reducing pain in the rainbow trout, Oncorhynchus mykiss". Applied Animal Behaviour Science. 133 (3): 265–274. doi:10.1016/j.applanim.2011.06.009.
{{cite journal}}
: CS1 maint: multiple names: authors list (link) - ^ Wolkers, C.P.B., Junior, B.A., Menescal-de-Oliveira, L. and Hoffmann, A. (2013). "Stress-induced antinociception in fish reversed by naloxone". PloS one. 8 (7): e71175. doi:10.1371/journal.pone.0071175.
{{cite journal}}
: CS1 maint: multiple names: authors list (link) CS1 maint: unflagged free DOI (link) - ^ Steenbergen, P.J. and Bardine, N. (2014). "Antinociceptive effects of buprenorphine in zebrafish larvae: An alternative for rodent models to study pain and nociception?". Applied Animal Behaviour Science. 152: 92–99. doi:10.1016/j.applanim.2013.12.001.
{{cite journal}}
: CS1 maint: multiple names: authors list (link) - Ehrensing, R.H., Michell, G.F. and Kastin, A.J. (1982). "Similar antagonism of morphine analgesia by MIF-1 and naloxone in Carassius auratus". Pharmacology Biochemistry and Behavior. 17 (4): 757–761. doi:10.1016/0091-3057(82)90358-6.
{{cite journal}}
: CS1 maint: multiple names: authors list (link) - Chervova, L.S. and Lapshin, D.N. (2000). "Opioid modulation of pain threshold in fish". Doklady Biological Sciences. 375 (1): 590–591.
{{cite journal}}
: CS1 maint: multiple names: authors list (link) - ^ "Physiological and behavioural responses to noxious stimuli in the Atlantic cod (Gadus morhua)". PLOS one. 2014. doi:10.1371/journal.pone.0100150.
{{cite journal}}
: Unknown parameter|authors=
ignored (help)CS1 maint: unflagged free DOI (link) - Grandin, T. (2015). "Chapter 2 - The importance of measurement to improve the welfare of livestock, poultry, and fish.". In Grandin T. (ed.). Improving Animal Welfare: A Practical Approach.
- Reilly, S.C., Quinn, J.P., Cossins, A.R. and Sneddon, L.U. (2008). "Behavioural analysis of a nociceptive event in fish: Comparisons between three species demonstrate specific responses". Applied Animal Behaviour Science. 114 (1): 248–259.
{{cite journal}}
: CS1 maint: multiple names: authors list (link) - Roques, J.A.C., Abbink, W., Geurds, F., van de Vis, H. and Flik, G. (2010). "Tailfin clipping, a painful procedure: Studies on Nile tilapia and common carp". Physiology & Behavior. 101 (4): 533–540.
{{cite journal}}
: CS1 maint: multiple names: authors list (link) - ^ Sneddon, L.U. (2009). "Pain and distress in fish". Institute for Laboratory Animals Journal. 50 (4): 338–342.
- Dunayer, Joan, "Fish: Sensitivity Beyond the Captor's Grasp," The Animals' Agenda, July/August 1991, pp. 12-18
- "Animal Cognition". Retrieved September 15, 2015.
- Dunlop, R., Millsopp, S. and Laming, P. (2006). "Avoidance learning in goldfish (Carassius auratus) and trout (Oncorhynchus mykiss) and implications for pain perception". Applied Animal Behaviour Science. 97 (2): 255–271. doi:10.1016/j.applanim.2005.06.018.
{{cite journal}}
: CS1 maint: multiple names: authors list (link) - ^ Millsopp, S. and Laming, P. (2007). "Trade-offs between feeding and shock avoidance in goldfish (Carassius auratus)". Applied Animal Behaviour Science. 113: 247–254. doi:10.1016/j.applanim.2007.11.004.
{{cite journal}}
: CS1 maint: multiple names: authors list (link) - ^ Sneddon L.U., Braithwaite V.A. and Gentle M.J. (2003). "Novel object test: Examining pain and fear in the rainbow trout". The Journal of Pain. 4: 431–440. doi:10.1067/S1526-5900(03)00717-X.
- Ashley, P.J., Ringrose, S., Edwards, K.L., Wallington, E., McCrohan, C.R. and Sneddon, L.U. (2009). "Effect of noxious stimulation upon antipredator responses and dominance status in rainbow trout". Animal Behaviour. 77 (2): 403–410. doi:10.1016/j.anbehav.2008.10.015.
{{cite journal}}
: CS1 maint: multiple names: authors list (link) - Broom, D.M. (2001). "Evolution of pain" (PDF). Vlaams Diergeneeskundig Tijdschrift. 70 (1): 17–21.
- Cooke, S.J. and Sneddon L.U. (2007). "Animal welfare perspectives on recreational angling". Applied Animal Behaviour Science. 104: 176–198. doi:10.1016/j.applanim.2006.09.002.
- Leake, J. (March 14, 2004). "Anglers to Face RSPCA Check". The Sunday Times. Retrieved September 15, 2015.
- Sneddon, L.U. (2006). "Ethics and welfare: Pain perception in fish". Bull. Eur. Assoc. Fish. Pathol. 26 (1): 6–10.
- "Animals (Scientific Procedures) Act 1986" (PDF). Home Office (UK). Retrieved September 23, 2015.
- "Animal Welfare Act 2006". UK Government. 2006. Retrieved September 25, 2015.
- "Animals in research". neavs. Retrieved September 25, 2015.
- Henriksen, S., Vaagland, H., Sundt-Hansen, L., May, R. and Fjellheim, A. (2003). "Consequences of pain perception in fish for catch and release, aquaculture and commercial fisheries" (PDF).
{{cite web}}
: CS1 maint: multiple names: authors list (link) - Rose, J.D. (2002). "The neurobehavioral nature of fishes and the question of awareness and pain" (PDF). Reviews in Fisheries Science. 10 (1): 1–38.
- Rose, J.D. (2002). "Do fish feel pain?". Retrieved September 27, 2007.
- Rose, J.D. (2003) A Critique of the paper: "Do fish have nociceptors: Evidence for the evolution of a vertebrate sensory system" In: Information Resources on Fish Welfare 1970-2003, Animal Welfare Information Resources No. 20. H. E. Erickson, Ed., U. S. Department of Agriculture, Beltsville, MD. Pp. 49-51.
- "Fish lack the brains to feel pain, says the latest school of thought". The Telegraph. February 10, 2003.
- Rose, J.D. (2007). "Anthropomorphism and 'mental welfare' of fishes". Diseases of Aquatic Organisms. 75: 139–154.
- Sneddon, L.U. (August 28, 2012). "Pain perception in fish: Why critics cannot accept the scientific evidence for fish pain" (PDF).
{{cite journal}}
: Cite journal requires|journal=
(help) - Sneddon, L.U. (2012). "Pain perception in fish: Evidence and implications for the use of fish". Journal of Consciousness Studies. 18: 209–229.
- Newby, N.C. and Stevens, E.D. (2008). "The effects of the acetic acid "pain" test on feeding, swimming and respiratory responses of rainbow trout (Oncorhynchus mykiss)". Applied Animal Behavior Science. 114 (1): 260–269. doi:10.1016/j.applanim.2007.12.006.
{{cite journal}}
: CS1 maint: multiple names: authors list (link) - Sneddon, L.U. (2009). "The effects of the acetic acid "pain" test on feeding, swimming, and respiratory responses of rainbow trout (Oncorhynchus mykiss): A critique on Newby and Stevens (2008)". Applied Animal Behaviour Science. 116 (1): 96–97.
- Newby, N.C. and Stevens, E.D. (2009). "The effects of the acetic acid "pain" test on feeding, swimming, and respiratory responses of rainbow trout (Oncorhynchus mykiss): A critique on Newby and Stevens (2008) — response". Applied Animal Behaviour Science. 116 (1): 97–99.
{{cite journal}}
: CS1 maint: multiple names: authors list (link) - ^ "Fish may actually feel pain and react to it much like humans". Purdue University. April 29, 2009.
Animal cognition | |
---|---|
Cognition | |
Intelligence | |
Pain | |
Relation to brain | |
Fish | |||||
---|---|---|---|---|---|
About fish | ![]() | ||||
Anatomy and physiology | |||||
Sensory systems | |||||
Reproduction | |||||
Locomotion | |||||
Other behaviour | |||||
By habitat | |||||
Other types | |||||
Commerce |
| ||||
Major groups | |||||
Lists | |||||