Revision as of 20:27, 18 May 2007 editReddi (talk | contribs)Autopatrolled, Extended confirmed users58,350 editsNo edit summary← Previous edit | Latest revision as of 09:55, 6 October 2024 edit undoTrasheater Midir (talk | contribs)Extended confirmed users3,670 edits →External links | ||
(626 intermediate revisions by more than 100 users not shown) | |||
Line 1: | Line 1: | ||
{{Short description|Subfield of astronomy that studies celestial objects at radio frequencies}} | |||
'''Radio ]''' is the study of celestial phenomena through measurement of ] emitted by physical processes occurring in space. Radio waves have a much greater wavelength than ]. In order to receive signals with large ], radio astronomy requires a large antenna or an array of smaller antennas working together (for example, the ]). Most of these ''']s''' use a parabolic dish to reflect the waves to a receiver which detects and amplifies the signal into usable data. This allows astronomers to see a region of the radio sky. If they take multiple scans of overlapping strips of the sky they can piece together an image (']'). Radio astronomy is a relatively new field of astronomical research that still has much more to be discovered. | |||
] ], a radio ] in ], ]]] | |||
'''Radio astronomy''' is a subfield of ] that studies ] at ]. The first detection of radio waves from an astronomical object was in 1933, when ] at ] reported radiation coming from the ]. Subsequent observations have identified a number of different sources of radio emission. These include ]s and ], as well as entirely new classes of objects, such as ], ]s, ]s, and ]. The discovery of the ], regarded as evidence for the ], was made through radio astronomy. | |||
Radio astronomy is conducted using large ] referred to as ]s, that are either used singularly, or with multiple linked telescopes utilizing the techniques of ] and ]. The use of interferometry allows radio astronomy to achieve high ], as the resolving power of an interferometer is set by the distance between its components, rather than the size of its components. | |||
== Astronomical sources == | |||
] at the ]. Just like in the visible, at low radio frequencies the sky is dominated by small bright sources, but the sources are typically active galaxies and supernova remnants rather than stars.]] | |||
Radio astronomy differs from '']'' in that the former is a passive observation (i.e., receiving only) and the latter an active one (transmitting and receiving). | |||
Radio astronomy has led to substantial increases in astronomical knowledge, particularly with the discovery of several classes of new objects, including ]s, ]s and ]. This is because radio astronomy allows us to see things that are not detectable in optical astronomy. Such objects represent some of the most extreme and energetic physical processes in the universe. | |||
== History == | |||
Radio astronomy is also partly responsible for the idea that ] is an important component of our universe; radio measurements of the rotation of ] suggest that there is much more mass in galaxies than has been directly observed (see ]). The ] was also first detected using radio telescopes. However, radio telescopes have also been used to investigate objects much closer to home, including observations of the ] and solar activity, and radar mapping of the ]. | |||
] and his rotating ] (early 1930s) in ], the world's first radio telescope, which was used to discover radio emissions from the ]]] | |||
Before Jansky observed the Milky Way in the 1930s, physicists speculated that radio waves could be observed from astronomical sources. In the 1860s, ]'s ] had shown that ] is associated with ] and ], and could exist at any ]. Several attempts were made to detect radio emission from the ] including an experiment by German astrophysicists ] and ] in 1896 and a centimeter wave radiation apparatus set up by ] between 1897 and 1900. These attempts were unable to detect any emission due to technical limitations of the instruments. The discovery of the radio reflecting ] in 1902, led physicists to conclude that the layer would bounce any astronomical radio transmission back into space, making them undetectable.<ref>{{cite web | url = http://www.nrao.edu/whatisra/hist_prehist.shtml | title = Pre-History of Radio Astronomy | author = F. Ghigo | publisher = ] | access-date = 2010-04-09 | archive-date = 2020-06-15 | archive-url = https://web.archive.org/web/20200615213814/http://www.nrao.edu/whatisra/hist_prehist.shtml | url-status = live }}</ref> | |||
] made the discovery of the first astronomical radio source ] in the early 1930s. As a newly hired radio engineer with ], he was assigned the task to investigate static that might interfere with ] transatlantic voice transmissions. Using a large ], Jansky noticed that his ] pen-and-paper recording system kept recording a persistent repeating signal or "hiss" of unknown origin. Since the signal peaked about every 24 hours, Jansky first suspected the source of the interference was the ] crossing the view of his directional antenna. Continued analysis, however, showed that the source was not following the 24-hour daily cycle of the Sun exactly, but instead repeating on a cycle of 23 hours and 56 minutes. Jansky discussed the puzzling phenomena with his friend, astrophysicist Albert Melvin Skellett, who pointed out that the observed time between the signal peaks was the exact length of a ]; the time it took for "fixed" astronomical objects, such as a star, to pass in front of the antenna every time the Earth rotated.<ref name="bookrags.com">{{cite book | url = http://www.bookrags.com/biography/karl-jansky-wsd/ | title = World of Scientific Discovery on Karl Jansky | access-date = 2010-04-09 | archive-date = 2012-01-21 | archive-url = https://web.archive.org/web/20120121112928/http://www.bookrags.com/biography/karl-jansky-wsd/ | url-status = live }}</ref> By comparing his observations with optical astronomical maps, Jansky eventually concluded that the radiation source peaked when his antenna was aimed at the densest part of the ] in the ] of ].<ref>{{cite journal | doi = 10.1038/132066a0 | author = Jansky, Karl G. | title = Radio waves from outside the solar system | journal = Nature | volume = 132 | issue = 3323 | page = 66 | date = 1933 | bibcode = 1933Natur.132...66J| s2cid = 4063838 | doi-access = free }}</ref> | |||
Jansky announced his discovery at a meeting in Washington, D.C., in April 1933 and the field of radio astronomy was born.<ref name="aas">{{cite web |last1=Hirshfeld |first1=Alan |title=Karl Jansky and the Discovery of Cosmic Radio Waves |url=https://aas.org/posts/news/2018/07/month-astronomical-history-1 |publisher=American Astronomical Society |access-date=21 September 2021 |date=2018 |quote=In April 1933, closing in on nearly two years of study, Jansky read his breakthrough paper, “Electrical Disturbances Apparently of Extraterrestrial Origin,” before a meeting of the International Scientific Radio Union in Washington, DC. The strongest of the extraterrestrial waves, he found, emanate from a region in Sagittarius centered around right ascension 18 hours and declination — 20 degrees — in other words, from the direction of the galactic center. Jansky’s discovery made the front page of the New York Times on 5 May 1933, and the field of radio astronomy was born. |archive-date=29 September 2021 |archive-url=https://web.archive.org/web/20210929185905/https://aas.org/posts/news/2018/07/month-astronomical-history-1 |url-status=live }}</ref> In October 1933, his discovery was published in a journal article entitled "Electrical disturbances apparently of extraterrestrial origin" in the '']''.<ref>{{cite journal |last=Jansky |first=Karl Guthe |author-link=Karl Guthe Jansky |date=October 1933 |title=Electrical disturbances apparently of extraterrestrial origin |journal=Proc. IRE |volume=21 |issue=10 |page=1387 |doi=10.1109/JRPROC.1933.227458 }} Reprinted 65 years later as {{cite journal |last=Jansky |first=Karl Guthe |author-link=Karl Guthe Jansky |title=Electrical disturbances apparently of extraterrestrial origin |doi=10.1109/JPROC.1998.681378 |journal=] |volume=86 |issue=7 |date=July 1998 |pages=1510–1515|s2cid=47549559 }} along with an explanatory preface by W.A. Imbriale, {{doi-inline|10.1109/JPROC.1998.681377|Introduction To "Electrical Disturbances Apparently Of Extraterrestrial Origin"}}.</ref> Jansky concluded that since the Sun (and therefore other stars) were not large emitters of radio noise, the strange radio interference may be generated by interstellar gas and dust in the galaxy, in particular, by "thermal agitation of charged particles."<ref name="bookrags.com"/><ref>{{cite journal |last=Jansky |first=Karl Guthe |author-link=Karl Guthe Jansky |date=October 1935 |title=A note on the source of interstellar interference |journal=Proc. IRE |volume=23 |issue=10 |page=1158|doi=10.1109/JRPROC.1935.227275 |s2cid=51632813 }}</ref> (Jansky's peak radio source, one of the brightest in the sky, was designated ] in the 1950s and was later hypothesized to be emitted by ] in a strong magnetic field. Current thinking is that these are ions in orbit around a massive ] at the center of the galaxy at a point now designated as Sagittarius A*. The asterisk indicates that the particles at Sagittarius A are ionized.)<ref>{{cite book |title=Relativity, Astrophysics and Cosmology: Volume 1 |last=Belusević |first=R. |year=2008 |url=https://books.google.com/books?id=WeICTHIxP2MC&pg=PA163|page=163 |publisher=Wiley-VCH |isbn=978-3-527-40764-4}}</ref><ref>{{Cite book |last=Kambič |first=B. |title=Viewing the Constellations with Binoculars |date=6 October 2009 |url=https://books.google.com/books?id=3vxLNPNHOcwC&pg=PA131|pages=131–133 |publisher=] |isbn=978-0-387-85355-0}}</ref><ref>{{cite journal | last1 = Gillessen | first1 = S. | last2 = Eisenhauer | first2 = F. | last3 = Trippe | first3 = S. |display-authors=etal | year = 2009 | title = Monitoring Stellar Orbits around the Massive Black Hole in the Galactic Center | journal = The Astrophysical Journal | volume = 692 | issue = 2| pages = 1075–1109 | doi = 10.1088/0004-637X/692/2/1075 | arxiv=0810.4674 | bibcode=2009ApJ...692.1075G| s2cid = 1431308 }}</ref><ref>{{cite journal | last1 = Brown | first1 = R.L. | year = 1982 | title = Precessing jets in Sagittarius A – Gas dynamics in the central parsec of the galaxy | bibcode = 1982ApJ...262..110B| journal = Astrophysical Journal | volume = 262 | pages = 110–119 | doi = 10.1086/160401| doi-access = free }}</ref> | |||
After 1935, Jansky wanted to investigate the radio waves from the Milky Way in further detail, but Bell Labs reassigned him to another project, so he did no further work in the field of astronomy. His pioneering efforts in the field of radio astronomy have been recognized by the naming of the fundamental unit of ], the ] (Jy), after him.<ref name="aps">{{cite web |title=This Month in Physics History May 5, 1933: The New York Times Covers Discovery of Cosmic Radio Waves |url=https://www.aps.org/publications/apsnews/201505/physicshistory.cfm |website=aps.org |publisher=American Physical Society (May 2015) Volume 24, Number 5 |access-date=21 September 2021 |quote=Jansky died in 1950 at the age of 44, the result of a massive stroke stemming from his kidney disease. When that first 1933 paper was reprinted in Proceedings of the IEEE in 1984, the editors noted that Jansky’s work would mostly likely have won a Nobel prize, had the scientist not died so young. Today the “jansky” is the unit of measurement for radio wave intensity (flux density). |archive-date=14 September 2021 |archive-url=https://web.archive.org/web/20210914000424/https://www.aps.org/publications/apsnews/201505/physicshistory.cfm |url-status=live }}</ref> | |||
]'s Antenna at ], world's first parabolic radio telescope]] | |||
] was inspired by Jansky's work, and built a parabolic radio telescope 9m in diameter in his backyard in 1937. He began by repeating Jansky's observations, and then conducted the first sky survey in the radio frequencies.<ref>{{cite web | url = http://www.nrao.edu/whatisra/hist_reber.shtml | title = Grote Reber | access-date = 2010-04-09 | archive-date = 2020-08-07 | archive-url = https://web.archive.org/web/20200807095454/https://www.nrao.edu/whatisra/hist_reber.shtml | url-status = live }}</ref> On February 27, 1942, ], a ] research officer, made the first detection of radio waves emitted by the Sun.<ref>{{cite book |last=Hey |first=J.S. |title=Radio Universe |edition=2nd |publisher=] |year=1975 |isbn= 978-0080187617 }}</ref> Later that year ],<ref>{{cite journal |last=Southworth |first=G.C. |year=1945 |title=Microwave radiation from the Sun |journal=Journal of the Franklin Institute |volume=239 |issue=4 |pages=285–297 |doi=10.1016/0016-0032(45)90163-3}}</ref> at ] like Jansky, also detected radiowaves from the Sun. Both researchers were bound by wartime security surrounding radar, so Reber, who was not, published his 1944 findings first.<ref>{{cite journal|last1=Kellerman|first1=K. I.|title=Grote Reber's Observations on Cosmic Static|journal=Astrophysical Journal|date= 1999|volume=525C|page=371 |bibcode=1999ApJ...525C.371K}}</ref> Several other people independently discovered solar radio waves, including ] in ]<ref>{{cite journal |last=Schott |first=E. |year=1947 |title=175 MHz-Strahlung der Sonne |journal=Physikalische Blätter |volume=3 |issue=5 |pages=159–160 |doi=10.1002/phbl.19470030508 |language=de|doi-access=free }}</ref> and ] working on ].<ref>{{cite book |last1=Alexander |first1=F.E.S. |date=1945 |title=Long Wave Solar Radiation |publisher=], Radio Development Laboratory }}</ref><ref>{{cite book |last1=Alexander |first1=F.E.S. |date=1945 |title=Report of the Investigation of the "Norfolk Island Effect" |publisher=], Radio Development Laboratory |bibcode=1945rdlr.book.....A }}</ref><ref>{{cite journal |last1=Alexander |first1=F.E.S. |date=1946 |title=The Sun's radio energy |journal=Radio & Electronics |volume=1 |issue=1 |pages=16–17}} (see {{Webarchive|url=https://archive.today/20160723215554/http://nlnzcat.natlib.govt.nz/vwebv/holdingsInfo?bibId=405978 |date=2016-07-23 }}.)</ref><ref name=Orchiston>{{cite book |last=Orchiston |first=W. |year=2005 |chapter=Dr Elizabeth Alexander: First Female Radio Astronomer |title=The New Astronomy: Opening the Electromagnetic Window and Expanding Our View of Planet Earth |series=Astrophysics and Space Science Library |volume=334 |pages=71–92 |doi=10.1007/1-4020-3724-4_5 |isbn=978-1-4020-3723-8}}</ref> | |||
] first recognised evidence of a ], in 1967 (exhibited at ]) ]] | |||
At ], where ionospheric research had taken place during ], ] along with other members of the ] that had carried out wartime research into ], created a radiophysics group at the university where radio wave emissions from the Sun were observed and studied. | |||
This early research soon branched out into the observation of other celestial radio sources and interferometry techniques were pioneered to isolate the angular source of the detected emissions. ] and ] at the ] developed the technique of Earth-rotation ]. The radio astronomy group in Cambridge went on to found the ] near Cambridge in the 1950s. During the late 1960s and early 1970s, as computers (such as the ]) became capable of handling the computationally intensive ] inversions required, they used aperture synthesis to create a 'One-Mile' and later a '5 km' effective aperture using the One-Mile and Ryle telescopes, respectively. They used the ] to map the radio sky, producing the ] (2C) and ] (3C) Cambridge Catalogues of Radio Sources.<ref>{{cite web|url=http://www.phy.cam.ac.uk/history/years/radioast.php|archive-url=https://web.archive.org/web/20131110022209/http://www.phy.cam.ac.uk/history/years/radioast.php|archive-date=2013-11-10|title=Radio Astronomy|publisher=Cambridge University: Department of Physics}}</ref> | |||
==Techniques== | |||
]) of various ]s of electromagnetic radiation]] | |||
Radio astronomers use different techniques to observe objects in the radio spectrum. Instruments may simply be pointed at an energetic radio source to analyze its emission. To "image" a region of the sky in more detail, multiple overlapping scans can be recorded and pieced together in a ] image. The type of instrument used depends on the strength of the signal and the amount of detail needed. | |||
Observations from the ]'s surface are limited to wavelengths that can pass through the atmosphere. At low frequencies or long wavelengths, transmission is limited by the ], which reflects waves with frequencies less than its characteristic ]. ] ] interferes with radio astronomy at higher frequencies, which has led to building radio observatories that conduct observations at ] wavelengths at very high and dry sites, in order to minimize the water vapor content in the line of sight. Finally, transmitting devices on Earth may cause ]. Because of this, many radio observatories are built at remote places. | |||
===Radio telescopes=== | |||
{{Main|Radio telescope}} | |||
Radio telescopes may need to be extremely large in order to receive signals with low ]. Also since ] is a function of the diameter of the "]" in proportion to the wavelength of the electromagnetic radiation being observed, '']s'' have to be much larger in comparison to their ] counterparts. For example, a 1-meter diameter optical telescope is two million times bigger than the wavelength of light observed giving it a resolution of roughly 0.3 ]s, whereas a radio telescope "dish" many times that size may, depending on the wavelength observed, only be able to resolve an object the size of the full moon (30 minutes of arc). | |||
===Radio interferometry=== | |||
{{main|Astronomical interferometry}} | |||
{{see also|Radio telescope#Radio interferometry}} | |||
] (ALMA), many antennas linked together in a radio interferometer]] | |||
] ] (]), a radio image of same galaxy using interferometry (], VLA), and an image of the center section (VLBA) using a Very Long Baseline Array (Global VLBI) consisting of antennas in the US, Germany, Italy, Finland, Sweden and Spain. The jet of particles is suspected to be powered by a ] in the center of the galaxy.]] | |||
The difficulty in achieving high resolutions with single radio telescopes led to radio ], developed by British radio astronomer ] and Australian engineer, radiophysicist, and radio astronomer ] and ] in 1946. The first use of a radio interferometer for an astronomical observation was carried out by Payne-Scott, Pawsey and ] on 26 January 1946 using a ''single'' converted radar antenna (broadside array) at ] near ]. This group used the principle of a sea-cliff interferometer in which the antenna (formerly a World War II radar) observed the Sun at sunrise with interference arising from the direct radiation from the Sun and the reflected radiation from the sea. With this baseline of almost 200 meters, the authors determined that the solar radiation during the burst phase was much smaller than the solar disk and arose from a region associated with a large ] group. The Australia group laid out the principles of ] in a ground-breaking paper published in 1947. The use of a sea-cliff ] had been demonstrated by numerous groups in Australia, Iran and the UK during World War II, who had observed interference fringes (the direct radar return radiation and the reflected signal from the sea) from incoming aircraft. | |||
The Cambridge group of Ryle and Vonberg observed the Sun at 175 MHz for the first time in mid July 1946 with a Michelson interferometer consisting of two radio antennas with spacings of some tens of meters up to 240 meters. They showed that the radio radiation was smaller than 10 ] in size and also detected circular polarization in the Type I bursts. Two other groups had also detected circular polarization at about the same time (] in Australia and ] with ] in the UK). | |||
Modern ] consist of widely separated radio telescopes observing the same object that are connected together using ], ], ], or other type of ]. This not only increases the total signal collected, it can also be used in a process called ] to vastly increase resolution. This technique works by superposing ("]") the signal ]s from the different telescopes on the principle that ]s that coincide with the same ] will add to each other while two waves that have opposite phases will cancel each other out. This creates a combined telescope that is the size of the antennas furthest apart in the array. In order to produce a high quality image, a large number of different separations between different telescopes are required (the projected separation between any two telescopes as seen from the radio source is called a "baseline") – as many different baselines as possible are required in order to get a good quality image. For example, the ] has 27 telescopes giving 351 independent baselines at once. | |||
====Very-long-baseline interferometry==== | |||
{{main|Very-long-baseline interferometry}} | |||
Beginning in the 1970s, improvements in the stability of radio telescope receivers permitted telescopes from all over the world (and even in Earth orbit) to be combined to perform ]. Instead of physically connecting the antennas, data received at each antenna is paired with timing information, usually from a local ], and then stored for later analysis on magnetic tape or hard disk. At that later time, the data is correlated with data from other antennas similarly recorded, to produce the resulting image. Using this method it is possible to synthesise an antenna that is effectively the size of the Earth. The large distances between the telescopes enable very high angular resolutions to be achieved, much greater in fact than in any other field of astronomy. At the highest frequencies, synthesised beams less than 1 ] are possible. | |||
The pre-eminent VLBI arrays operating today are the ] (with telescopes located across North America) and the ] (telescopes in Europe, China, South Africa and Puerto Rico). Each array usually operates separately, but occasional projects are observed together producing increased sensitivity. This is referred to as Global VLBI. There are also a VLBI networks, operating in Australia and New Zealand called the LBA (Long Baseline Array),<ref>{{Cite web| url=http://www.atnf.csiro.au/vlbi/| title=VLBI at the ATNF| date=7 December 2016| access-date=16 June 2015| archive-date=1 May 2021| archive-url=https://web.archive.org/web/20210501051105/https://www.atnf.csiro.au/vlbi/| url-status=live}}</ref> and arrays in Japan, China and South Korea which observe together to form the East-Asian VLBI Network (EAVN).<ref>{{Cite web | url=http://www.astro.sci.yamaguchi-u.ac.jp/eavn/index.html | title=East Asia VLBI Network and Asia Pacific Telescope | access-date=2015-06-16 | archive-date=2021-04-28 | archive-url=https://web.archive.org/web/20210428080543/http://astro.sci.yamaguchi-u.ac.jp/eavn/index.html | url-status=live }}</ref> | |||
Since its inception, recording data onto hard media was the only way to bring the data recorded at each telescope together for later correlation. However, the availability today of worldwide, high-bandwidth networks makes it possible to do VLBI in real time. This technique (referred to as e-VLBI) was originally pioneered in Japan, and more recently adopted in Australia and in Europe by the EVN (European VLBI Network) who perform an increasing number of scientific e-VLBI projects per year.<ref>{{Cite web |url=http://www.innovations-report.com/html/reports/physics_astronomy/report-25117.html |title=A technological breakthrough for radio astronomy – Astronomical observations via high-speed data link<!-- Bot generated title --> |date=26 January 2004 |access-date=2008-07-22 |archive-date=2008-12-03 |archive-url=https://web.archive.org/web/20081203145055/http://www.innovations-report.com/html/reports/physics_astronomy/report-25117.html |url-status=live }}</ref> | |||
==Astronomical sources== | |||
{{Main|Astronomical radio source}} {{See also|Radio object with continuous optical spectrum}} | |||
].]] | |||
Radio astronomy has led to substantial increases in astronomical knowledge, particularly with the discovery of several classes of new objects, including ]s, ]s<ref name=Shields>{{cite journal|last1=Shields|first1=Gregory A.|title=A brief history of AGN|journal=The Publications of the Astronomical Society of the Pacific|date=1999|volume=111|issue=760|pages=661–678|access-date=3 October 2014|url=http://ned.ipac.caltech.edu/level5/Sept04/Shields/Shields3.html|doi=10.1086/316378|arxiv=astro-ph/9903401|bibcode=1999PASP..111..661S|s2cid=18953602|archive-date=12 September 2009|archive-url=https://web.archive.org/web/20090912025415/http://nedwww.ipac.caltech.edu/level5/Sept04/Shields/Shields3.html|url-status=live}}</ref> and ]. This is because radio astronomy allows us to see things that are not detectable in optical astronomy. Such objects represent some of the most extreme and energetic physical processes in the universe. | |||
The ] was also first detected using radio telescopes. However, radio telescopes have also been used to investigate objects much closer to home, including observations of the ] and solar activity, and radar mapping of the ]. | |||
Other sources include: | Other sources include: | ||
* ] | |||
* ] and ]s have jets of charged particles which emit ] | |||
* ] | |||
* Merging ] often show diffuse radio emission | |||
* ], the ] of the ], with one portion ] thought to be a radio wave emitting ] | |||
* ]s can also show diffuse radio emission | |||
* ] and ]s have jets of charged particles which emit ] | |||
* The ] is ] radio emission | |||
* Merging ]s often show diffuse radio emission<ref>{{cite web |url=http://www.arcetri.astro.it/~buttery/thesis/node69.html |title=Conclusion |access-date=2006-03-29 |url-status=dead |archive-url=https://web.archive.org/web/20060128231925/http://www.arcetri.astro.it/~buttery/thesis/node69.html |archive-date=2006-01-28 }}</ref> | |||
* ]s can also show diffuse radio emission; ]s are a type of supernova remnant that shows highly synchronous emission. | |||
* The ] is ] radio/microwave emission | |||
Earth's radio signal is mostly natural and stronger than for example Jupiter's, but is produced by Earth's ]s and bounces at the ] back into space.<ref name="Geophysical Institute 1983 r818">{{cite web | title=The Earth is a Strong Radio Source even without Man's Tinkering | website=Geophysical Institute | date=June 23, 1983 | url=https://www.gi.alaska.edu/alaska-science-forum/earth-strong-radio-source-even-without-mans-tinkering | access-date=May 2, 2024}}</ref> | |||
== Observational techniques == | |||
Radio telescopes can now be found all over the world (see ]). Most are designed for ] radiation. Widely separated telescopes are often combined using a technique called ] in order to obtain observations with much higher resolution than could be obtained using a single receiver. Initially telescopes within a few kilometers of each other were combined (see, for example, the ]), but since the 1970s telescopes from all over the world (and even in Earth orbit) have been combined to perform ]. | |||
==International regulation== | |||
The United States government has established an institution to conduct radio astronomy research in the US, titled the ] (commonly abbreviated as NRAO). This institution controls various radio telescopes around the United States included the world's largest fully mobile radio telescope, the ]. The United States government has also set aside a ] for radio astronomy research centered around ]. As a result, Green Bank is now the home of ]'s primary facility. | |||
], ]]] | |||
], US]] | |||
] | |||
'''Radio astronomy service''' (also: ''radio astronomy radiocommunication service'') is, according to Article 1.58 of the ] (ITU) ] (RR),<ref>ITU Radio Regulations, Section IV. Radio Stations and Systems – Article 1.58, definition: '' radio astronomy service / radio astronomy radiocommunication service''</ref> defined as "A ] involving the use of radio astronomy". Subject of this radiocommunication service is to receive ]s transmitted by ] or celestial objects. | |||
''See also:'' | |||
*] | |||
*] | |||
===Frequency allocation=== | |||
== Historical development == | |||
The allocation of radio frequencies is provided according to ''Article 5'' of the ITU Radio Regulations (edition 2012).<ref>''ITU Radio Regulations, CHAPTER II – Frequencies, ARTICLE 5 Frequency allocations, Section IV – Table of Frequency Allocations''</ref> | |||
{{seealso|History of astronomical interferometry}} | |||
Modern radio astronomy started with a ] discovery by ], an engineer with ], in the early 1930s. Jansky was investigating static that interfered with ] voice transmissions using a turntable mounted 100 ft. by 20 ft. ] working at a ] of 20.5 ] (wavelength about 14.6 meters). By rotating the antenna the direction of a received "static" could be pinpointed. A small shed to the side of the antenna housed an ] pen-and-paper recording system. After sorting out signals from nearby and distant thunderstorms, Jansky continued to investigate a faint steady hiss of unknown origin. Jansky finally determined that the signal repeated on a cycle of 23 hours and 56 minutes. This four-minute lag is typical of an astronomical source "fixed" on the ] rotating in sync with ]. By comparing his observations with optical astronomical maps, Jansky concluded that the radiation was coming from the ] and was strongest in the direction of the center the galaxy, in the ] of ]. | |||
In order to improve harmonisation in spectrum utilisation, the majority of service-allocations stipulated in this document were incorporated in national Tables of Frequency Allocations and Utilisations which is with-in the responsibility of the appropriate national administration. The allocation might be primary, secondary, exclusive, and shared. | |||
] helped pioneer radio astronomy when he built the first parabolic "dish" radio telescope (9m in diameter) in 1937. He was instrumental in repeating Karl Guthe Jansky's pioneering but somewhat simple work, and went on to conduct the first sky survey in the radio frequencies. On ] ], ], a ] research officer, helped progress radio astronomy further, when he discovered that the sun emitted radio waves. After ], substantial improvements in radio astronomy technology were made by astronomers in Europe, Australia and the United States, and the field of radio astronomy began to blossom. | |||
*primary allocation: is indicated by writing in capital letters (see example below) | |||
*secondary allocation: is indicated by small letters | |||
*exclusive or shared utilization: is within the responsibility of administrations | |||
In line to the appropriate ] the frequency bands are allocated (primary or secondary) to the ''radio astronomy service'' as follows. | |||
One of the most notable developments came in 1946 with the introduction of the technique called ] where many radio telescopes are combined in a large array to achieve much higher ]. ]'s ] obtained a ] for this and later ] work<ref>] 158 pp 339 1946</ref>. The ] interferometer was also developed independently in 1946 by ]'s group at the CSIR, (later ]) in Sydney<ref>] 157 pp 158 1946</ref>. In the early 1950s the ] mapped the radio sky to produce the famous ] and ] surveys of radio sources. Two issues, one astronomical and one technical, dominated the research in Cambridge, from the late 1940's for more than thirty years. What was the nature of the discrete radio sources, or `radio stars'? Where were they, what were they, what were their properties, how many were there, how did they work and what was their significance in the Universe? Of parallel importance was the puzzle of how to devise new kinds of radio telescope which would elucidate these astronomical questions. | |||
===Earliest observation=== | |||
{{main|Teslascope}} | |||
{| class=wikitable | |||
In 1899, the eccentric ], in accord with many other plans of his, planned to build a tower in a experimental station at Colorado topped by a copper ball that he would turn into a sensitive ].<ref>Eric Brus, Richard Golob (1990). The Almanac of Science and Technology: What's New and What's Known. 530 pages. Page 52.</ref><ref>Margaret Cheney, Robert Uth, Jim Glenn (1999). Tesla, Master of Lightning. 184 pages. Page 95.</ref> While investigating atmospheric electricity in 1900, Tesla noted repetitive signals that he deduced must be coming from a non-terrestrial source. Although Tesla mistook this to be radio communication from intelligent beings living on ] or ]<ref>Tesla, Nikola, "''''". Collier's Weekly, February 19, 1901. (EarlyRadioHistory.us)</ref> it may have been the earliest observation of an astronomical radio source (A 1996 analysis indicated Tesla may have been observing ] ] signals).<ref>Corum, K. L., J. F. Corum, "''''".</ref> | |||
|- bgcolor="#CCCCCC" align="center" | |||
|align="center" colspan="3"| '''Allocation to services''' | |||
|- align="center" | |||
| Region 1 | |||
| Region 2 | |||
| Region 3 | |||
|- | |||
|colspan="3"|13 360–13 410 '''kHz''' FIXED<br /> | |||
::::: '''RADIO ASTRONOMY''' | |||
|- | |||
|colspan="3"|25 550–25 650 '''RADIO ASTRONOMY''' | |||
|- | |||
|colspan="3"|37.5–38.25 '''MHz''' FIXED<br /> | |||
::::: MOBILE<br />'''Radio astronomy''' | |||
|- | |||
|colspan="3"|322–328.6 FIXED<br /> | |||
:::: MOBILE<br />'''RADIO ASTRONOMY''' | |||
|- | |||
|colspan="3"|406.1–410 FIXED<br /> | |||
:::: MOBILE except aeronautical mobile<br />'''RADIO ASTRONOMY''' | |||
|- | |||
|colspan="3"|1 400–1 427 EARTH EXPLORATION-SATELLITE (passive)<br /> | |||
:::: '''RADIO ASTRONOMY'''<br />SPACE RESEARCH (passive) | |||
|- | |||
|colspan="1"|1 610.6–1 613.8<br /> | |||
MOBILE-SATELLITE <br /> | |||
:(Earth-to-space) | |||
'''RADIO ASTRONOMY'''<br />AERONAUTICAL <br /> | |||
:RADIONAVIGATION | |||
<br /> | |||
<br /> | |||
|colspan="1"|1 610.6–1 613.8<br /> | |||
MOBILE-SATELLITE <br /> | |||
:(Earth-to-space) | |||
'''RADIO ASTRONOMY''' <br />AERONAUTICAL <br /> | |||
:RADIONAVIGATION | |||
RADIODETERMINATION-<br /> | |||
:SATELLITE (Earth-to-space) | |||
|colspan="1"|1 610.6–1 613.8<br /> | |||
MOBILE-SATELLITE <br /> | |||
:(Earth-to-space) | |||
'''RADIO ASTRONOMY''' <br />AERONAUTICAL <br /> | |||
:RADIONAVIGATION | |||
Radiodetermination-<br /> | |||
:satellite (Earth-to-space) | |||
|- | |||
|colspan="3"|10.6–10.68 '''GHz''' '''RADIO ASTRONOMY''' and other services | |||
|- | |||
|colspan="3"|10.68–10.7 '''RADIO ASTRONOMY''' and other services | |||
|- | |||
|colspan="3"|14.47–14.5 '''RADIO ASTRONOMY''' and other services | |||
|- | |||
|colspan="3"|15.35–15.4 '''RADIO ASTRONOMY''' and other services | |||
|- | |||
|colspan="3"|22.21–22.5 '''RADIO ASTRONOMY''' and other services | |||
|- | |||
|colspan="3"|23.6–24 '''RADIO ASTRONOMY''' and other services | |||
|- | |||
|colspan="3"|31.3–31.5 '''RADIO ASTRONOMY''' and other services | |||
|- | |||
|} | |||
== |
==See also== | ||
{{Portal|Radio|Astronomy}} | |||
<references/> | |||
* ] | |||
* ] | |||
* ] | |||
* ] | |||
* ] | |||
* ] | |||
* ] | |||
* ] (radio instrument on the ''Juno'' Jupiter orbiter) | |||
* ] | |||
* ] | |||
{{clear right}} | |||
== References == | |||
{{Reflist}} | |||
== Further reading == | |||
{{Refbegin}} | |||
; Journals | |||
* {{cite journal |author=Gart Westerhout |title=The early history of radio astronomy |journal=] |volume=189 |year = 1972 |issue=1 |pages = 211–218 |doi = 10.1111/j.1749-6632.1972.tb12724.x |bibcode = 1972NYASA.198..211W |s2cid=56034495 }} | |||
* {{cite journal |author=Hendrik Christoffel van de Hulst |year=1945 |title=Radiostraling uit het wereldruim. II. Herkomst der radiogolven |journal = Nederlands Tijdschrift voor Natuurkunde |volume=11 |pages=210–221 |language = nl }} | |||
; Books | |||
* ] ''The Invisible Universe: The Story of Radio Astronomy'' Springer 2015 | |||
* Bruno Bertotti (ed.), ''Modern Cosmology in Retrospect''. Cambridge University Press 1990. | |||
* James J. Condon, et al.: ''Essential Radio Astronomy.'' Princeton University Press, Princeton 2016, {{ISBN|9780691137797}}. | |||
* Robin Michael Green, ''Spherical Astronomy''. Cambridge University Press, 1985. | |||
* Raymond Haynes, Roslynn Haynes, and Richard McGee, ''Explorers of the Southern Sky: A History of Australian Astronomy''. Cambridge University Press 1996. | |||
* J.S. Hey, ''The Evolution of Radio Astronomy.'' Neale Watson Academic, 1973. | |||
* David L. Jauncey, ''Radio Astronomy and Cosmology.'' Springer 1977. | |||
* ], ''Introduction to Radio Astronomy''. 1967. | |||
* Jobn D. Kraus, Martt; E. Tiuri, and Antti V. Räisänen, ''Radio Astronomy'', 2nd ed, Cygnus-Quasar Books, 1986. | |||
* Albrecht Krüger, ''Introduction to Solar Radio Astronomy and Radio Physics.'' Springer 1979. | |||
* David P.D. Munns, ''A Single Sky: How an International Community Forged the Science of Radio Astronomy.'' Cambridge, MA: MIT Press, 2013. | |||
* Allan A. Needell, ''Science, Cold War and American State: Lloyd V. Berkner and the Balance of Professional Ideals''. Routledge, 2000. | |||
* Joseph Lade Pawsey and Ronald Newbold Bracewell, ''Radio Astronomy.'' Clarendon Press, 1955. | |||
* Kristen Rohlfs, Thomas L Wilson, ''Tools of Radio Astronomy''. Springer 2003. | |||
* D.T. Wilkinson and P.J.E. Peebles, ''Serendipitous Discoveries in Radio Astronomy.'' Green Bank, WV: National Radio Astronomy Observatory, 1983. | |||
* Woodruff T. Sullivan III, ''The Early Years of Radio Astronomy: Reflections Fifty Years after Jansky's Discovery.'' Cambridge, England: Cambridge University Press, 1984. | |||
* Woodruff T. Sullivan III, ''Cosmic Noise: A History of Early Radio Astronomy.'' Cambridge University Press, 2009. | |||
* Woodruff T. Sullivan III, ''Classics in Radio Astronomy''. Reidel Publishing Company, Dordrecht, 1982. | |||
{{Refend}} | |||
== External links == | == External links == | ||
{{Commons category|Radio astronomy}} | |||
* | |||
* | |||
* - a history of ] radio astronomy | |||
* |
* * | ||
* – a brief history from ] ] | |||
* - , ] | |||
* | |||
* | |||
* - a brief history from ] ] | * | ||
* | |||
* Hanes, Dave, "''Physics 014: The Course Notes, ''". Astronomy Group and Department of Physics, Queen's University. 2000-2001. | |||
* {{Webarchive|url=https://web.archive.org/web/20110404054247/http://www.levanda.co.il/ronny/RadioAstronomy_SignalProcessing.html |date=2011-04-04 }} | |||
* – Radioastrolab | |||
* | |||
{{Clear}} | |||
{{EMSpectrum}} | |||
{{Radio-astronomy |state = uncollapsed }} | |||
{{Astronomy navbox}} | |||
{{Authority control}} | |||
{{DEFAULTSORT:Radio Astronomy}} | |||
] | |||
] | ] | ||
] | ] | ||
] | ] | ||
] | |||
] | |||
] | |||
] | |||
] | |||
] | |||
] | |||
] | |||
] | |||
] | |||
] | |||
] | |||
] | |||
] | |||
] | |||
] | |||
] | |||
] | |||
] | |||
] | |||
] |
Latest revision as of 09:55, 6 October 2024
Subfield of astronomy that studies celestial objects at radio frequencies
Radio astronomy is a subfield of astronomy that studies celestial objects at radio frequencies. The first detection of radio waves from an astronomical object was in 1933, when Karl Jansky at Bell Telephone Laboratories reported radiation coming from the Milky Way. Subsequent observations have identified a number of different sources of radio emission. These include stars and galaxies, as well as entirely new classes of objects, such as radio galaxies, quasars, pulsars, and masers. The discovery of the cosmic microwave background radiation, regarded as evidence for the Big Bang theory, was made through radio astronomy.
Radio astronomy is conducted using large radio antennas referred to as radio telescopes, that are either used singularly, or with multiple linked telescopes utilizing the techniques of radio interferometry and aperture synthesis. The use of interferometry allows radio astronomy to achieve high angular resolution, as the resolving power of an interferometer is set by the distance between its components, rather than the size of its components.
Radio astronomy differs from radar astronomy in that the former is a passive observation (i.e., receiving only) and the latter an active one (transmitting and receiving).
History

Before Jansky observed the Milky Way in the 1930s, physicists speculated that radio waves could be observed from astronomical sources. In the 1860s, James Clerk Maxwell's equations had shown that electromagnetic radiation is associated with electricity and magnetism, and could exist at any wavelength. Several attempts were made to detect radio emission from the Sun including an experiment by German astrophysicists Johannes Wilsing and Julius Scheiner in 1896 and a centimeter wave radiation apparatus set up by Oliver Lodge between 1897 and 1900. These attempts were unable to detect any emission due to technical limitations of the instruments. The discovery of the radio reflecting ionosphere in 1902, led physicists to conclude that the layer would bounce any astronomical radio transmission back into space, making them undetectable.
Karl Jansky made the discovery of the first astronomical radio source serendipitously in the early 1930s. As a newly hired radio engineer with Bell Telephone Laboratories, he was assigned the task to investigate static that might interfere with short wave transatlantic voice transmissions. Using a large directional antenna, Jansky noticed that his analog pen-and-paper recording system kept recording a persistent repeating signal or "hiss" of unknown origin. Since the signal peaked about every 24 hours, Jansky first suspected the source of the interference was the Sun crossing the view of his directional antenna. Continued analysis, however, showed that the source was not following the 24-hour daily cycle of the Sun exactly, but instead repeating on a cycle of 23 hours and 56 minutes. Jansky discussed the puzzling phenomena with his friend, astrophysicist Albert Melvin Skellett, who pointed out that the observed time between the signal peaks was the exact length of a sidereal day; the time it took for "fixed" astronomical objects, such as a star, to pass in front of the antenna every time the Earth rotated. By comparing his observations with optical astronomical maps, Jansky eventually concluded that the radiation source peaked when his antenna was aimed at the densest part of the Milky Way in the constellation of Sagittarius.
Jansky announced his discovery at a meeting in Washington, D.C., in April 1933 and the field of radio astronomy was born. In October 1933, his discovery was published in a journal article entitled "Electrical disturbances apparently of extraterrestrial origin" in the Proceedings of the Institute of Radio Engineers. Jansky concluded that since the Sun (and therefore other stars) were not large emitters of radio noise, the strange radio interference may be generated by interstellar gas and dust in the galaxy, in particular, by "thermal agitation of charged particles." (Jansky's peak radio source, one of the brightest in the sky, was designated Sagittarius A in the 1950s and was later hypothesized to be emitted by electrons in a strong magnetic field. Current thinking is that these are ions in orbit around a massive black hole at the center of the galaxy at a point now designated as Sagittarius A*. The asterisk indicates that the particles at Sagittarius A are ionized.)
After 1935, Jansky wanted to investigate the radio waves from the Milky Way in further detail, but Bell Labs reassigned him to another project, so he did no further work in the field of astronomy. His pioneering efforts in the field of radio astronomy have been recognized by the naming of the fundamental unit of flux density, the jansky (Jy), after him.
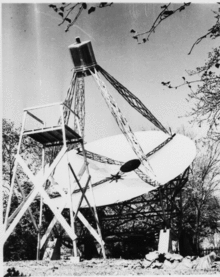
Grote Reber was inspired by Jansky's work, and built a parabolic radio telescope 9m in diameter in his backyard in 1937. He began by repeating Jansky's observations, and then conducted the first sky survey in the radio frequencies. On February 27, 1942, James Stanley Hey, a British Army research officer, made the first detection of radio waves emitted by the Sun. Later that year George Clark Southworth, at Bell Labs like Jansky, also detected radiowaves from the Sun. Both researchers were bound by wartime security surrounding radar, so Reber, who was not, published his 1944 findings first. Several other people independently discovered solar radio waves, including E. Schott in Denmark and Elizabeth Alexander working on Norfolk Island.

At Cambridge University, where ionospheric research had taken place during World War II, J. A. Ratcliffe along with other members of the Telecommunications Research Establishment that had carried out wartime research into radar, created a radiophysics group at the university where radio wave emissions from the Sun were observed and studied. This early research soon branched out into the observation of other celestial radio sources and interferometry techniques were pioneered to isolate the angular source of the detected emissions. Martin Ryle and Antony Hewish at the Cavendish Astrophysics Group developed the technique of Earth-rotation aperture synthesis. The radio astronomy group in Cambridge went on to found the Mullard Radio Astronomy Observatory near Cambridge in the 1950s. During the late 1960s and early 1970s, as computers (such as the Titan) became capable of handling the computationally intensive Fourier transform inversions required, they used aperture synthesis to create a 'One-Mile' and later a '5 km' effective aperture using the One-Mile and Ryle telescopes, respectively. They used the Cambridge Interferometer to map the radio sky, producing the Second (2C) and Third (3C) Cambridge Catalogues of Radio Sources.
Techniques
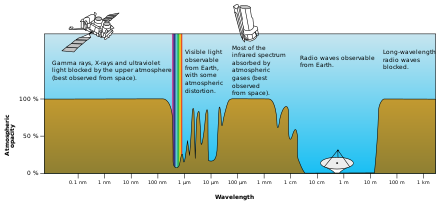
Radio astronomers use different techniques to observe objects in the radio spectrum. Instruments may simply be pointed at an energetic radio source to analyze its emission. To "image" a region of the sky in more detail, multiple overlapping scans can be recorded and pieced together in a mosaic image. The type of instrument used depends on the strength of the signal and the amount of detail needed.
Observations from the Earth's surface are limited to wavelengths that can pass through the atmosphere. At low frequencies or long wavelengths, transmission is limited by the ionosphere, which reflects waves with frequencies less than its characteristic plasma frequency. Water vapor interferes with radio astronomy at higher frequencies, which has led to building radio observatories that conduct observations at millimeter wavelengths at very high and dry sites, in order to minimize the water vapor content in the line of sight. Finally, transmitting devices on Earth may cause radio-frequency interference. Because of this, many radio observatories are built at remote places.
Radio telescopes
Main article: Radio telescopeRadio telescopes may need to be extremely large in order to receive signals with low signal-to-noise ratio. Also since angular resolution is a function of the diameter of the "objective" in proportion to the wavelength of the electromagnetic radiation being observed, radio telescopes have to be much larger in comparison to their optical counterparts. For example, a 1-meter diameter optical telescope is two million times bigger than the wavelength of light observed giving it a resolution of roughly 0.3 arc seconds, whereas a radio telescope "dish" many times that size may, depending on the wavelength observed, only be able to resolve an object the size of the full moon (30 minutes of arc).
Radio interferometry
Main article: Astronomical interferometry See also: Radio telescope § Radio interferometry


The difficulty in achieving high resolutions with single radio telescopes led to radio interferometry, developed by British radio astronomer Martin Ryle and Australian engineer, radiophysicist, and radio astronomer Joseph Lade Pawsey and Ruby Payne-Scott in 1946. The first use of a radio interferometer for an astronomical observation was carried out by Payne-Scott, Pawsey and Lindsay McCready on 26 January 1946 using a single converted radar antenna (broadside array) at 200 MHz near Sydney, Australia. This group used the principle of a sea-cliff interferometer in which the antenna (formerly a World War II radar) observed the Sun at sunrise with interference arising from the direct radiation from the Sun and the reflected radiation from the sea. With this baseline of almost 200 meters, the authors determined that the solar radiation during the burst phase was much smaller than the solar disk and arose from a region associated with a large sunspot group. The Australia group laid out the principles of aperture synthesis in a ground-breaking paper published in 1947. The use of a sea-cliff interferometer had been demonstrated by numerous groups in Australia, Iran and the UK during World War II, who had observed interference fringes (the direct radar return radiation and the reflected signal from the sea) from incoming aircraft.
The Cambridge group of Ryle and Vonberg observed the Sun at 175 MHz for the first time in mid July 1946 with a Michelson interferometer consisting of two radio antennas with spacings of some tens of meters up to 240 meters. They showed that the radio radiation was smaller than 10 arc minutes in size and also detected circular polarization in the Type I bursts. Two other groups had also detected circular polarization at about the same time (David Martyn in Australia and Edward Appleton with James Stanley Hey in the UK).
Modern radio interferometers consist of widely separated radio telescopes observing the same object that are connected together using coaxial cable, waveguide, optical fiber, or other type of transmission line. This not only increases the total signal collected, it can also be used in a process called aperture synthesis to vastly increase resolution. This technique works by superposing ("interfering") the signal waves from the different telescopes on the principle that waves that coincide with the same phase will add to each other while two waves that have opposite phases will cancel each other out. This creates a combined telescope that is the size of the antennas furthest apart in the array. In order to produce a high quality image, a large number of different separations between different telescopes are required (the projected separation between any two telescopes as seen from the radio source is called a "baseline") – as many different baselines as possible are required in order to get a good quality image. For example, the Very Large Array has 27 telescopes giving 351 independent baselines at once.
Very-long-baseline interferometry
Main article: Very-long-baseline interferometryBeginning in the 1970s, improvements in the stability of radio telescope receivers permitted telescopes from all over the world (and even in Earth orbit) to be combined to perform very-long-baseline interferometry. Instead of physically connecting the antennas, data received at each antenna is paired with timing information, usually from a local atomic clock, and then stored for later analysis on magnetic tape or hard disk. At that later time, the data is correlated with data from other antennas similarly recorded, to produce the resulting image. Using this method it is possible to synthesise an antenna that is effectively the size of the Earth. The large distances between the telescopes enable very high angular resolutions to be achieved, much greater in fact than in any other field of astronomy. At the highest frequencies, synthesised beams less than 1 milliarcsecond are possible.
The pre-eminent VLBI arrays operating today are the Very Long Baseline Array (with telescopes located across North America) and the European VLBI Network (telescopes in Europe, China, South Africa and Puerto Rico). Each array usually operates separately, but occasional projects are observed together producing increased sensitivity. This is referred to as Global VLBI. There are also a VLBI networks, operating in Australia and New Zealand called the LBA (Long Baseline Array), and arrays in Japan, China and South Korea which observe together to form the East-Asian VLBI Network (EAVN).
Since its inception, recording data onto hard media was the only way to bring the data recorded at each telescope together for later correlation. However, the availability today of worldwide, high-bandwidth networks makes it possible to do VLBI in real time. This technique (referred to as e-VLBI) was originally pioneered in Japan, and more recently adopted in Australia and in Europe by the EVN (European VLBI Network) who perform an increasing number of scientific e-VLBI projects per year.
Astronomical sources
Main article: Astronomical radio source See also: Radio object with continuous optical spectrum
Radio astronomy has led to substantial increases in astronomical knowledge, particularly with the discovery of several classes of new objects, including pulsars, quasars and radio galaxies. This is because radio astronomy allows us to see things that are not detectable in optical astronomy. Such objects represent some of the most extreme and energetic physical processes in the universe.
The cosmic microwave background radiation was also first detected using radio telescopes. However, radio telescopes have also been used to investigate objects much closer to home, including observations of the Sun and solar activity, and radar mapping of the planets.
Other sources include:
- Sun
- Jupiter
- Sagittarius A, the Galactic Center of the Milky Way, with one portion Sagittarius A* thought to be a radio wave emitting supermassive black hole
- Active galactic nuclei and pulsars have jets of charged particles which emit synchrotron radiation
- Merging galaxy clusters often show diffuse radio emission
- Supernova remnants can also show diffuse radio emission; pulsars are a type of supernova remnant that shows highly synchronous emission.
- The cosmic microwave background is blackbody radio/microwave emission
Earth's radio signal is mostly natural and stronger than for example Jupiter's, but is produced by Earth's auroras and bounces at the ionosphere back into space.
International regulation
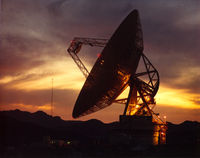

Radio astronomy service (also: radio astronomy radiocommunication service) is, according to Article 1.58 of the International Telecommunication Union's (ITU) Radio Regulations (RR), defined as "A radiocommunication service involving the use of radio astronomy". Subject of this radiocommunication service is to receive radio waves transmitted by astronomical or celestial objects.
Frequency allocation
The allocation of radio frequencies is provided according to Article 5 of the ITU Radio Regulations (edition 2012).
In order to improve harmonisation in spectrum utilisation, the majority of service-allocations stipulated in this document were incorporated in national Tables of Frequency Allocations and Utilisations which is with-in the responsibility of the appropriate national administration. The allocation might be primary, secondary, exclusive, and shared.
- primary allocation: is indicated by writing in capital letters (see example below)
- secondary allocation: is indicated by small letters
- exclusive or shared utilization: is within the responsibility of administrations
In line to the appropriate ITU Region the frequency bands are allocated (primary or secondary) to the radio astronomy service as follows.
Allocation to services | ||
Region 1 | Region 2 | Region 3 |
13 360–13 410 kHz FIXED
| ||
25 550–25 650 RADIO ASTRONOMY | ||
37.5–38.25 MHz FIXED
| ||
322–328.6 FIXED
| ||
406.1–410 FIXED
| ||
1 400–1 427 EARTH EXPLORATION-SATELLITE (passive)
| ||
1 610.6–1 613.8 MOBILE-SATELLITE
RADIO ASTRONOMY
|
1 610.6–1 613.8 MOBILE-SATELLITE
RADIO ASTRONOMY
RADIODETERMINATION-
|
1 610.6–1 613.8 MOBILE-SATELLITE
RADIO ASTRONOMY
Radiodetermination-
|
10.6–10.68 GHz RADIO ASTRONOMY and other services | ||
10.68–10.7 RADIO ASTRONOMY and other services | ||
14.47–14.5 RADIO ASTRONOMY and other services | ||
15.35–15.4 RADIO ASTRONOMY and other services | ||
22.21–22.5 RADIO ASTRONOMY and other services | ||
23.6–24 RADIO ASTRONOMY and other services | ||
31.3–31.5 RADIO ASTRONOMY and other services |
See also
- Atacama Large Millimeter Array
- Channel 37
- Gamma-ray astronomy
- Infrared astronomy
- Radar astronomy
- Time smearing
- X-ray astronomy
- Waves (Juno) (radio instrument on the Juno Jupiter orbiter)
- Radio Galaxy Zoo
- Würzburg radar#Post-war use in astronomy
References
- F. Ghigo. "Pre-History of Radio Astronomy". National Radio Astronomy Observatory. Archived from the original on 2020-06-15. Retrieved 2010-04-09.
- ^ World of Scientific Discovery on Karl Jansky. Archived from the original on 2012-01-21. Retrieved 2010-04-09.
- Jansky, Karl G. (1933). "Radio waves from outside the solar system". Nature. 132 (3323): 66. Bibcode:1933Natur.132...66J. doi:10.1038/132066a0. S2CID 4063838.
- Hirshfeld, Alan (2018). "Karl Jansky and the Discovery of Cosmic Radio Waves". American Astronomical Society. Archived from the original on 29 September 2021. Retrieved 21 September 2021.
In April 1933, closing in on nearly two years of study, Jansky read his breakthrough paper, "Electrical Disturbances Apparently of Extraterrestrial Origin," before a meeting of the International Scientific Radio Union in Washington, DC. The strongest of the extraterrestrial waves, he found, emanate from a region in Sagittarius centered around right ascension 18 hours and declination — 20 degrees — in other words, from the direction of the galactic center. Jansky's discovery made the front page of the New York Times on 5 May 1933, and the field of radio astronomy was born.
- Jansky, Karl Guthe (October 1933). "Electrical disturbances apparently of extraterrestrial origin". Proc. IRE. 21 (10): 1387. doi:10.1109/JRPROC.1933.227458. Reprinted 65 years later as Jansky, Karl Guthe (July 1998). "Electrical disturbances apparently of extraterrestrial origin". Proc. IEEE. 86 (7): 1510–1515. doi:10.1109/JPROC.1998.681378. S2CID 47549559. along with an explanatory preface by W.A. Imbriale, Introduction To "Electrical Disturbances Apparently Of Extraterrestrial Origin" .
- Jansky, Karl Guthe (October 1935). "A note on the source of interstellar interference". Proc. IRE. 23 (10): 1158. doi:10.1109/JRPROC.1935.227275. S2CID 51632813.
- Belusević, R. (2008). Relativity, Astrophysics and Cosmology: Volume 1. Wiley-VCH. p. 163. ISBN 978-3-527-40764-4.
- Kambič, B. (6 October 2009). Viewing the Constellations with Binoculars. Springer. pp. 131–133. ISBN 978-0-387-85355-0.
- Gillessen, S.; Eisenhauer, F.; Trippe, S.; et al. (2009). "Monitoring Stellar Orbits around the Massive Black Hole in the Galactic Center". The Astrophysical Journal. 692 (2): 1075–1109. arXiv:0810.4674. Bibcode:2009ApJ...692.1075G. doi:10.1088/0004-637X/692/2/1075. S2CID 1431308.
- Brown, R.L. (1982). "Precessing jets in Sagittarius A – Gas dynamics in the central parsec of the galaxy". Astrophysical Journal. 262: 110–119. Bibcode:1982ApJ...262..110B. doi:10.1086/160401.
- "This Month in Physics History May 5, 1933: The New York Times Covers Discovery of Cosmic Radio Waves". aps.org. American Physical Society (May 2015) Volume 24, Number 5. Archived from the original on 14 September 2021. Retrieved 21 September 2021.
Jansky died in 1950 at the age of 44, the result of a massive stroke stemming from his kidney disease. When that first 1933 paper was reprinted in Proceedings of the IEEE in 1984, the editors noted that Jansky's work would mostly likely have won a Nobel prize, had the scientist not died so young. Today the "jansky" is the unit of measurement for radio wave intensity (flux density).
- "Grote Reber". Archived from the original on 2020-08-07. Retrieved 2010-04-09.
- Hey, J.S. (1975). Radio Universe (2nd ed.). Pergamon Press. ISBN 978-0080187617.
- Southworth, G.C. (1945). "Microwave radiation from the Sun". Journal of the Franklin Institute. 239 (4): 285–297. doi:10.1016/0016-0032(45)90163-3.
- Kellerman, K. I. (1999). "Grote Reber's Observations on Cosmic Static". Astrophysical Journal. 525C: 371. Bibcode:1999ApJ...525C.371K.
- Schott, E. (1947). "175 MHz-Strahlung der Sonne". Physikalische Blätter (in German). 3 (5): 159–160. doi:10.1002/phbl.19470030508.
- Alexander, F.E.S. (1945). Long Wave Solar Radiation. Department of Scientific and Industrial Research, Radio Development Laboratory.
- Alexander, F.E.S. (1945). Report of the Investigation of the "Norfolk Island Effect". Department of Scientific and Industrial Research, Radio Development Laboratory. Bibcode:1945rdlr.book.....A.
- Alexander, F.E.S. (1946). "The Sun's radio energy". Radio & Electronics. 1 (1): 16–17. (see R&E holdings at NLNZ Archived 2016-07-23 at archive.today.)
- Orchiston, W. (2005). "Dr Elizabeth Alexander: First Female Radio Astronomer". The New Astronomy: Opening the Electromagnetic Window and Expanding Our View of Planet Earth. Astrophysics and Space Science Library. Vol. 334. pp. 71–92. doi:10.1007/1-4020-3724-4_5. ISBN 978-1-4020-3723-8.
- "Radio Astronomy". Cambridge University: Department of Physics. Archived from the original on 2013-11-10.
- "VLBI at the ATNF". 7 December 2016. Archived from the original on 1 May 2021. Retrieved 16 June 2015.
- "East Asia VLBI Network and Asia Pacific Telescope". Archived from the original on 2021-04-28. Retrieved 2015-06-16.
- "A technological breakthrough for radio astronomy – Astronomical observations via high-speed data link". 26 January 2004. Archived from the original on 2008-12-03. Retrieved 2008-07-22.
- Shields, Gregory A. (1999). "A brief history of AGN". The Publications of the Astronomical Society of the Pacific. 111 (760): 661–678. arXiv:astro-ph/9903401. Bibcode:1999PASP..111..661S. doi:10.1086/316378. S2CID 18953602. Archived from the original on 12 September 2009. Retrieved 3 October 2014.
- "Conclusion". Archived from the original on 2006-01-28. Retrieved 2006-03-29.
- "The Earth is a Strong Radio Source even without Man's Tinkering". Geophysical Institute. June 23, 1983. Retrieved May 2, 2024.
- ITU Radio Regulations, Section IV. Radio Stations and Systems – Article 1.58, definition: radio astronomy service / radio astronomy radiocommunication service
- ITU Radio Regulations, CHAPTER II – Frequencies, ARTICLE 5 Frequency allocations, Section IV – Table of Frequency Allocations
Further reading
- Journals
- Gart Westerhout (1972). "The early history of radio astronomy". Annals of the New York Academy of Sciences. 189 (1): 211–218. Bibcode:1972NYASA.198..211W. doi:10.1111/j.1749-6632.1972.tb12724.x. S2CID 56034495.
- Hendrik Christoffel van de Hulst (1945). "Radiostraling uit het wereldruim. II. Herkomst der radiogolven". Nederlands Tijdschrift voor Natuurkunde (in Dutch). 11: 210–221.
- Books
- Gerrit Verschuur The Invisible Universe: The Story of Radio Astronomy Springer 2015
- Bruno Bertotti (ed.), Modern Cosmology in Retrospect. Cambridge University Press 1990.
- James J. Condon, et al.: Essential Radio Astronomy. Princeton University Press, Princeton 2016, ISBN 9780691137797.
- Robin Michael Green, Spherical Astronomy. Cambridge University Press, 1985.
- Raymond Haynes, Roslynn Haynes, and Richard McGee, Explorers of the Southern Sky: A History of Australian Astronomy. Cambridge University Press 1996.
- J.S. Hey, The Evolution of Radio Astronomy. Neale Watson Academic, 1973.
- David L. Jauncey, Radio Astronomy and Cosmology. Springer 1977.
- Roger Clifton Jennison, Introduction to Radio Astronomy. 1967.
- Jobn D. Kraus, Martt; E. Tiuri, and Antti V. Räisänen, Radio Astronomy, 2nd ed, Cygnus-Quasar Books, 1986.
- Albrecht Krüger, Introduction to Solar Radio Astronomy and Radio Physics. Springer 1979.
- David P.D. Munns, A Single Sky: How an International Community Forged the Science of Radio Astronomy. Cambridge, MA: MIT Press, 2013.
- Allan A. Needell, Science, Cold War and American State: Lloyd V. Berkner and the Balance of Professional Ideals. Routledge, 2000.
- Joseph Lade Pawsey and Ronald Newbold Bracewell, Radio Astronomy. Clarendon Press, 1955.
- Kristen Rohlfs, Thomas L Wilson, Tools of Radio Astronomy. Springer 2003.
- D.T. Wilkinson and P.J.E. Peebles, Serendipitous Discoveries in Radio Astronomy. Green Bank, WV: National Radio Astronomy Observatory, 1983.
- Woodruff T. Sullivan III, The Early Years of Radio Astronomy: Reflections Fifty Years after Jansky's Discovery. Cambridge, England: Cambridge University Press, 1984.
- Woodruff T. Sullivan III, Cosmic Noise: A History of Early Radio Astronomy. Cambridge University Press, 2009.
- Woodruff T. Sullivan III, Classics in Radio Astronomy. Reidel Publishing Company, Dordrecht, 1982.
External links
- nrao.edu National Radio Astronomy Observatory
- The History of Radio Astronomy * Reber Radio Telescope – National Park Services
- Radio Telescope Developed – a brief history from NASA Goddard Space Flight Center
- Society of Amateur Radio Astronomers
- Visualization of Radio Telescope Data Using Google Earth
- UnwantedEmissions.com A general reference for radio spectrum allocations, including radio astronomy.
- Improving Radio Astronomy Images by Array Processing Archived 2011-04-04 at the Wayback Machine
- What is Radio Astronomy – Radioastrolab
- PICTOR: A free-to-use radio telescope
Astronomy | |||||||||||
---|---|---|---|---|---|---|---|---|---|---|---|
Astronomy by |
| ||||||||||
Optical telescopes | |||||||||||
Related | |||||||||||