Revision as of 16:07, 24 February 2006 view sourceVsmith (talk | contribs)Administrators271,832 editsm Reverted edits by 151.188.0.231 (talk) to last version by Soumyasch← Previous edit | Latest revision as of 03:59, 19 November 2024 view source TumCool412 (talk | contribs)15 edits →Hazards: fix link | ||
Line 1: | Line 1: | ||
{{Short description|Molten rock expelled by a volcano during an eruption}} | |||
{{otheruses}} | |||
{{Redirect|Lava flow|the programming anti-pattern|Lava flow (programming)|other uses|Lava (disambiguation)}} | |||
{{wiktionarypar3|lava|Aa|pahoehoe}} | |||
{{sprotect|small=yes}} | |||
'''Lava''' is molten rock that a ] expels during an eruption. Lava, when first exuded from a volcanic vent, is a ] at very high ]: typically from 700°C to 1200°C (1300°F to 2200°F). Although the ] of lava is 100,000 times that of water, it can flow many miles before eventually cooling and solidifying. | |||
{{pp-move-indef}} | |||
{{Use British English|date=January 2022}} | |||
] volcano eruption in Iceland, 2023]] | |||
While still below the earth's surface, molten rock is termed ]. Lava solidifies to form ]. The term "'''lava flow'''" refers to the hardened formation, whereas the one still having molten rock associated, is called an "'''active lava flow'''". The word 'lava' comes from Italian, and is probably ultimately derived from the ] word ''labes'' which means a fall, slide, or sinking in. The first time it was used in connection with extruded magma was apparently in a short account written by ] on the eruption of ] which took place between ] and ] ]. In this he described "a flow of fiery lava" as an analogy to the flow of water and mud down the flanks of the volcano following heavy rain. | |||
'''Lava''' is molten or partially molten rock (]) that has been expelled from the interior of a ] (such as ]) or a ] onto its surface. Lava may be erupted at a ] or through a ] in the ], on land or underwater, usually at temperatures from {{convert|800|to|1200|C|F}}. The ] resulting from subsequent cooling is also often called ''lava''. | |||
] | |||
A '''lava flow''' is an outpouring of lava during an ]. (An ], by contrast, produces a mixture of ] and other fragments called ], not lava flows.) The ] of most lava is about that of ], roughly 10,000 to 100,000 times that of water. Even so, lava can flow great distances before cooling causes it to solidify, because lava exposed to air quickly develops a solid crust that insulates the remaining liquid lava, helping to keep it hot and inviscid enough to continue flowing.<ref>{{cite book |last1=Philpotts |first1=Anthony R. |last2=Ague |first2=Jay J. |title=Principles of igneous and metamorphic petrology |date=2009 |publisher=Cambridge University Press |location=Cambridge, UK |isbn=9780521880060 |pages=53–55 |edition=2nd}}</ref> | |||
== Lava composition and rheology == | |||
Igneous rocks, which form lava flows when erupted, can be classified into three chemical types; ], intermediate and ]. These classes are primarily chemical; however, the chemistry of a lava also tends to correlate with the magma temperature, its viscosity and its mode of eruption. In general, a lava's composition determines its behavior more than the temperature of its eruption. | |||
== Etymology == | |||
''''']''''' lavas such as ] and ] are often associated with ] eruptions, typically form ]s and sheeted flows, and are associated with ] surge deposits and ]s. Felsic lavas are extremely viscous. This is caused primarily by the chemistry of the magma, which is high in ], ], ], ], and ], forming a ]ised liquid rich in ] and ], which is thus much more sticky than other magma types. Felsic magmas can be erupted at temperatures as low as 650 to 750 degrees Celsius, although they can be hotter. | |||
The word ''lava'' comes from ] and is probably derived from the ] word ''labes'', which means a fall or slide.<ref>{{cite web|url=http://www.m-w.com/dictionary/lava |title=Lava |work=Merriam-Webster Online Dictionary |date=2012-08-31 |access-date=8 December 2013}}</ref><ref>{{cite web|url=http://dictionary.reference.com/browse/lava |title=Lava |publisher=Dictionary.reference.com |date=1994-12-07 |access-date=8 December 2013}}</ref> An early use of the word in connection with extrusion of magma from below the surface is found in a short account of the 1737 eruption of ], written by ], who described "a flow of fiery lava" as an analogy to the flow of water and mud down the flanks of the volcano (a ]) after heavy ].<ref>{{cite book |last1=Serao |first1=Francesco |title=Istoria dell' incendio del Vesuvio accaduto nel mese di maggio dell'anno MDCCXXXVII |date=1778 |publisher=Presso Il De Bonis |location=Naples |url=https://archive.org/details/istoriadellincen00sera/page/n3/mode/2up |access-date=6 August 2022}}</ref><ref>{{cite web |title=Vesuvius Erupts, 1738 |url=https://vulcan.lindahall.org/9.shtml |website=Linda Hall Library of Science, Engineering & Technology |access-date=6 August 2022}}</ref> | |||
== Properties of lava == | |||
'''''Intermediate''''' magmas are lower in aluminium and silica, and usually somewhat richer in ] and ]. Intermediate lavas form ] domes and sheeted flows, are also usually associated with strombolian eruptions, and form composite volcanoes. Poorer in aluminium and silica, and also hotter (in the range of 750 to 950 degrees Celsius), they tend to be less viscous, although not generally fluid. Greater temperatures tend to destroy polymerized bonds within the magma, promoting more fluid behaviour and also a greater tendency to form ]. Higher iron and magnesium tends to manifest as a darker ], and also occasionally ] or ] ]s. | |||
=== Composition === | |||
] | |||
Solidified lava on the ] is predominantly ]: mostly ]s, ]s, ], ]s, ]s, ]s and ].{{sfn|Philpotts|Ague|2009|p=19}} Rare nonsilicate lavas can be formed by local melting of nonsilicate mineral deposits<ref name="ChileIronOxideLava">{{cite journal | url=https://www.researchgate.net/publication/241044499 | title=Geological, Geographical and Legal Considerations for the Conservation of Unique Iron Oxide and Sulphur Flows at El Laco and Lastarria Volcanic Complexes, Central Andes, Northern Chile | author=Guijón, R. |author2=Henríquez, F. |author3=Naranjo, J.A. | journal=Geoheritage | year=2011 | volume=3 | issue=4 | pages=99–315 | doi=10.1007/s12371-011-0045-x| bibcode=2011Geohe...3..299G | s2cid=129179725 }}</ref> or by separation of a magma into ] silicate and nonsilicate liquid ].<ref name="IronOxidelava">{{cite journal | url=https://www.researchgate.net/publication/235632335 | title=Apatite–monazite relations in the Kiirunavaara magnetite–apatite ore, northern Sweden | author=Harlov, D.E. | journal=Chemical Geology | year=2002 | volume=191 | issue=1–3 | pages=47–72 | doi=10.1016/s0009-2541(02)00148-1| bibcode=2002ChGeo.191...47H |display-authors=etal}}</ref> | |||
==== Silicate lavas ==== | |||
''''']''''' lavas are typified by ], which is generally erupted at temperatures in excess of 950 degrees Celsius. Basaltic magma is highest in iron and magnesium, and has relatively lower aluminium and silica, which taken together reduces the degree of polymerization within the melt. Due to the higher temperatures, viscosities can fall to relatively low levels, although this is still thousands of times more viscous than water. | |||
Silicate lavas are molten mixtures dominated by ] and ], the ], with smaller quantities of ], ], ], ], ], and ] and minor amounts of many other elements.{{sfn|Philpotts|Ague|2009|p=19}} ]s routinely express the composition of a silicate lava in terms of the weight or ] fraction of the oxides of the major elements (other than oxygen) present in the lava.{{sfn|Philpotts|Ague|2009|pp=132-133}} | |||
The low degree of polymerization and high temperatures favor chemical diffusion, so it is common to see large, well-formed phenocrysts within mafic lavas. Basalt volcanoes tend to form ], as the fluid magma tends to form thin, widely distributed flows. | |||
The silica component dominates the physical behavior of silicate magmas. Silicon ions in lava strongly bind to four oxygen ions in a tetrahedral arrangement. If an oxygen ion is bound to two silicon ions in the melt, it is described as a bridging oxygen, and lava with many clumps or chains of silicon ions connected by bridging oxygen ions is described as partially polymerized. Aluminium in combination with alkali metal oxides (sodium and potassium) also tends to polymerize the lava.{{sfn|Philpotts|Ague|2009|p=25}} Other ], such as ferrous iron, calcium, and magnesium, bond much more weakly to oxygen and reduce the tendency to polymerize.<ref>{{cite book |last1=Schmincke |first1=Hans-Ulrich |title=Volcanism |date=2003 |publisher=Springer |location=Berlin |isbn=9783540436508 |page=38}}</ref> Partial polymerization makes the lava viscous, so lava high in silica is much more viscous than lava low in silica.{{sfn|Philpotts|Ague|2009|p=25}} | |||
''''']''''' lavas such as ] and high-magnesian magmas which form ] take the composition and temperatures of eruptions to the extreme. Most if not all ultramafic lavas are no younger than the ], with a few ultramafic magmas known from the ]. No modern komatiite lavas are known, as the Earth's mantle has cooled too much to produce highly magnesian magmas. | |||
<br>Komatiites contain greater than 18% magnesium oxide, and are considered to have erupted at temperatures of 1600°C. At this temperature there is no polymerization of the mineral compounds, creating a superfluid liquid with the viscosity as low as that of water. | |||
Because of the role of silica in determining viscosity and because many other properties of a lava (such as its temperature) are observed to correlate with silica content, silicate lavas are divided into four chemical types based on silica content: ], ], ], and ].<ref>{{cite book |last1=Casq |first1=R.A.F. |url=https://archive.org/details/volcanicsuccessi0000casr/page/528/mode/2up |title=Volcanic Successions |last2=Wright |first2=J.V. |date=1987 |publisher=Unwin Hyman Inc |isbn=978-0-04-552022-0 |page=528 |url-access=registration}}</ref> | |||
===Lava Behavior=== | |||
The viscosity of lava is important because it determines how the lava will behave. Highly viscous lava shows the following behaviors: | |||
*It tends to flow slowly, clog, and form semi-solid blocks which resist flow | |||
*It tends to entrap gasses, which form bubbles within the rock as it rises to the surface | |||
*It correlates with explosive or ] eruptions and is associated with ] and ] flows | |||
===== Felsic lava ===== | |||
Highly viscous lavas do not usually flow as liquid, and usually form explosive fragmental ash and ] deposits. However, a degassed viscous lava or one which erupts somewhat hotter than usual may form a lava flow. Viscous lavas have two forms of non-pyroclastic eruptions, '''''lava domes''''' and '''''sheeted flows'''''. | |||
''Felsic'' or ] lavas have a silica content greater than 63%. They include ] and ] lavas. With such a high silica content, these lavas are extremely viscous, ranging from 10<sup>8</sup> ] (10<sup>5</sup> Pa⋅s) for hot rhyolite lava at {{cvt|1200|C||}} to 10<sup>11</sup> cP (10<sup>8</sup> Pa⋅s) for cool rhyolite lava at {{cvt|800|C||}}.{{sfn|Philpotts|Ague|2009|p=23}} For comparison, water has a viscosity of about 1 cP (0.001 Pa⋅s). Because of this very high viscosity, felsic lavas usually erupt explosively to produce ] (fragmental) deposits. However, rhyolite lavas occasionally erupt effusively to form ]s, ]s or "coulees" (which are thick, short lava flows).{{sfn|Philpotts|Ague|2009|pp=70-77}} The lavas typically fragment as they extrude, producing block lava flows. These often contain ].{{sfn|Schmincke|2003|p=132}} | |||
Felsic magmas can erupt at temperatures as low as {{convert|800|°C}}.{{sfn|Philpotts|Ague|2009|p=20}} Unusually hot (>950 °C; >1,740 °F) rhyolite lavas, however, may flow for distances of many tens of kilometres, such as in the ] of the northwestern United States.<ref>{{cite journal |last1=Bonnichsen |first1=B. |last2=Kauffman, D.F. |year=1987 |title=Physical features of rhyolite lava flows in the Snake River Plain volcanic province, southwestern Idaho |journal=Geological Society of America Special Paper |series=Geological Society of America Special Papers |volume=212 |pages=119–145|doi=10.1130/SPE212-p119 |isbn=0-8137-2212-8 }}</ref><!-- Rather an old source; are the Snake River flows still regarded as fluid flows and not ash flows?--> | |||
Conversely, lava with low viscosity shows the following behaviors: | |||
*It tends to flow easily, forming puddles, channels, and rivers of molten rock | |||
*It tends to easily release bubbling gasses as they are formed | |||
*Eruptions are rarely pyroclastic and are usually quiescent | |||
*Volcanoes tend to form as rifts, not steep cones | |||
===== Intermediate lava ===== | |||
There are three forms of low-viscosity lava flows: '''''‘a‘a''''', '''''pāhoehoe''''', and '''''pillow lava'''''. They are described in the following sections. | |||
''Intermediate'' or ] lavas contain 52% to 63% silica, and are lower in aluminium and usually somewhat richer in ] and ] than felsic lavas. Intermediate lavas form andesite domes and block lavas and may occur on steep ]es, such as in the ].{{sfn|Schmincke|2003|pp=21-24,132,143}} They are also commonly hotter than felsic lavas, in the range of {{convert|850|to|1100|°C}}. Because of their lower silica content and higher eruptive temperatures, they tend to be much less viscous, with a typical viscosity of 3.5 × 10<sup>6</sup> cP (3,500 Pa⋅s) at {{cvt|1200|C||}}. This is slightly greater than the viscosity of smooth ].{{sfn|Philpotts|Ague|2009|pp=23-611}} Intermediate lavas show a greater tendency to form ].<ref>{{cite journal |last1=Takeuchi |first1=Shingo |title=Preeruptive magma viscosity: An important measure of magma eruptibility |journal=Journal of Geophysical Research |date=5 October 2011 |volume=116 |issue=B10|pages=B10201 |doi=10.1029/2011JB008243| bibcode=2011JGRB..11610201T |doi-access=free }}</ref> Higher iron and magnesium tends to manifest as a darker ], including amphibole or pyroxene phenocrysts.{{sfn|Philpotts|Ague|2009|pp=1376-377}} | |||
===== Mafic lava ===== | |||
Of course, lavas also may contain many other components, sometimes including solid crystals of various minerals, fragments of exotic rocks known as ] and parts of its own solidified lava products. | |||
''Mafic'' or ] lavas are typified by relatively high magnesium oxide and iron oxide content (whose molecular formulas provide the consonants in mafic) and have a silica content limited to a range of 52% to 45%. They generally erupt at temperatures of {{convert|1100 to 1200|°C}} and at relatively low viscosities, around 10<sup>4</sup> to 10<sup>5</sup> cP (10 to 100 Pa⋅s). This is similar to the viscosity of ],{{sfn|Philpotts|Ague|2009|pp=23-25}} although it is still many orders of magnitude higher than that of water. Mafic lavas tend to produce low-profile ]es or ]s, because the less viscous lava can flow for long distances from the vent. The thickness of a solidified basaltic lava flow, particularly on a low slope, may be much greater than the thickness of the moving molten lava flow at any one time, because basaltic lavas may "inflate" by a continued supply of lava and its pressure on a solidified crust.{{sfn|Philpotts|Ague|2009|p=53-55, 59-64}} Most basaltic lavas are of {{okina}}a{{okina}}ā or pāhoehoe types, rather than block lavas. Underwater, they can form ], which are rather similar to entrail-type pahoehoe lavas on land.{{sfn|Schmincke|2003|pp=128-132}} | |||
=== |
===== Ultramafic lava ===== | ||
''Ultramafic'' lavas, such as ] and highly magnesian magmas that form ], take the composition and temperatures of eruptions to the extreme. All have a silica content under 45%. Komatiites contain over 18% magnesium oxide and are thought to have erupted at temperatures of {{convert|1600|°C}}. At this temperature there is practically no polymerization of the mineral compounds, creating a highly mobile liquid.<ref name="Condie1994">{{cite book | title=Archean Crustal Evolution | publisher=Elsevier | location=Amsterdam | editor=Condie, K.C.| year=1994 | chapter=Archean komatiites | author=Arndt, N.T. | page=19 | isbn=978-0-444-81621-4}}</ref> Viscosities of komatiite magmas are thought to have been as low as 100 to 1000 cP (0.1 to 1 Pa⋅s), similar to that of light motor oil.{{sfn|Philpotts|Ague|2009|p=23}} Most ultramafic lavas are no younger than the ], with a few ultramafic magmas known from the ] in Central America that are attributed to a hot ]. No modern komatiite lavas are known, as the ] has cooled too much to produce highly magnesian magmas.{{sfn|Philpotts|Ague|2009|pp=399-400}} | |||
] | |||
Cooling viscous lava often clogs a volcanic vent, allowing pressure behind the blockage to build; trapped gasses within the lava also add to the pressure, eventually producing cataclysmic explosions, ejecting great clouds of ] and gas, and producing ]. Most explosive eruptions tend to be followed by a quieter period of lava extrusion. | |||
===== Alkaline lavas ===== | |||
Sometimes as a volcano extrudes silicic lava, it forms an ''inflation dome'', gradually building up a large, pillow-like structure which cracks, fissures, and may release cooled chunks of rock and rubble. The top and side margins of an inflating lava dome tend to be covered in fragments of rock., ] and ash. | |||
Some silicate lavas have an elevated content of ]s (sodium and potassium), particularly in regions of ], areas overlying deeply ] ], or at intraplate ].{{sfn|Philpotts|Ague|2009|pp=139-148}} Their silica content can range from ultramafic (]s, ]s and ]s) to felsic (]s). They are more likely to be generated at greater depths in the mantle than subalkaline magmas.{{sfn|Philpotts|Ague|2009|pp=606-607}} Olivine ] lavas are both ultramafic and highly alkaline, and are thought to have come from much deeper in the ] of the ] than other lavas.<ref>{{cite web| url=http://gsc.nrcan.gc.ca/volcanoes/cat/feature_volcano_e.php |work=Catalogue of Canadian volcanoes| title=Stikine Volcanic Belt: Volcano Mountain|archive-url=https://web.archive.org/web/20090307140121/http://gsc.nrcan.gc.ca/volcanoes/cat/feature_volcano_e.php |archive-date=2009-03-07|access-date=23 November 2007}}</ref> | |||
{| style="border:0px solid black;" | |||
|+ | |||
!valign="top" | | |||
{| class="wikitable" style="text-align: left;" | |||
|+Examples of lava compositions (wt%){{sfn|Philpotts|Ague|2009|p=145}} | |||
! Component | |||
! ] | |||
! ] ] | |||
! ] | |||
! ] | |||
! ] | |||
|- | |||
| Silica |] | |||
| 39.7 | |||
| 46.4 | |||
| 53.8 | |||
| 60.0 | |||
| 73.2 | |||
|- | |||
| ] | |||
| 2.8 | |||
| 2.0 | |||
| 2.0 | |||
| 1.0 | |||
| 0.2 | |||
|- | |||
| ] | |||
| 11.4 | |||
| 8.5 | |||
| 13.9 | |||
| 16.0 | |||
| 14.0 | |||
|- | |||
| ] | |||
| 5.3 | |||
| 2.5 | |||
| 2.6 | |||
| 1.9 | |||
| 0.6 | |||
|- | |||
| ] | |||
| 8.2 | |||
| 9.8 | |||
| 9.3 | |||
| 6.2 | |||
| 1.7 | |||
|- | |||
| ] | |||
| 0.2 | |||
| 0.2 | |||
| 0.2 | |||
| 0.2 | |||
| 0.0 | |||
|- | |||
| ] | |||
| 12.1 | |||
| 20.8 | |||
| 4.1 | |||
| 3.9 | |||
| 0.4 | |||
|- | |||
| ] | |||
| 12.8 | |||
| 7.4 | |||
| 7.9 | |||
| 5.9 | |||
| 1.3 | |||
|- | |||
| ] | |||
| 3.8 | |||
| 1.6 | |||
| 3.0 | |||
| 3.9 | |||
| 3.9 | |||
|- | |||
| ] | |||
| 1.2 | |||
| 0.3 | |||
| 1.5 | |||
| 0.9 | |||
| 4.1 | |||
|- | |||
| ] | |||
| 0.9 | |||
| 0.2 | |||
| 0.4 | |||
| 0.2 | |||
| 0.0 | |||
|} | |||
! valign="top" | {{Pie chart | |||
| radius = 100 | |||
| thumb = | |||
| caption = Tholeiitic basalt lava | |||
| other = | |||
| label1 = SiO<sub>2</sub> | |||
| value1 = 53.8 | |||
| label2 = Al<sub>2</sub>O<sub>3</sub> | |||
| value2 =13.9 | |||
| label3 =FeO | |||
| value3 =9.3 | |||
| label4 =CaO | |||
| value4 =7.9 | |||
| label5 =MgO | |||
| value5 =4.1 | |||
| label6 =Na<sub>2</sub>O | |||
| value6 =3.0 | |||
| label7 = Fe<sub>2</sub>O<sub>3</sub> | |||
| value7=2.6 | |||
| label8 = TiO<sub>2</sub> | |||
| value8=2.0 | |||
| label9 = K<sub>2</sub>O | |||
| value9 = 1.5 | |||
| label10 = P<sub>2</sub>O<sub>5</sub> | |||
| value10 = 0.4 | |||
| label11 = MnO | |||
| value11 = 0.2 | |||
}} | |||
! valign="top" |{{Pie chart | |||
| radius = 100 | |||
| thumb = | |||
| caption = Rhyolite lava | |||
| other = | |||
| label1 = SiO<sub>2</sub> | |||
| value1 = 73.2 | |||
| label2 = Al<sub>2</sub>O<sub>3</sub> | |||
| value2 = 14 | |||
| label9 =K<sub>2</sub>O | |||
| value9 = 4.1 | |||
| label6 =Na<sub>2</sub>O | |||
| value6 = 3.9 | |||
| label3 =FeO | |||
| value3 = 1.7 | |||
| label4 = CaO | |||
| value4 = 1.3 | |||
| label7 = Fe<sub>2</sub>O<sub>3</sub> | |||
| value7= 0.6 | |||
| label5 = MgO | |||
| value5= 0.4 | |||
| label8 = TiO<sub>2</sub> | |||
| value8 = 0.2 | |||
| label10 = P<sub>2</sub>O<sub>5</sub> | |||
| value10 = 0. | |||
| label11 = MnO | |||
| value11 = 0. | |||
}} | |||
|} | |||
==== Non-silicate lavas ==== | |||
Examples of lava dome eruptions include the ] dome, and successive lava domes of ]. | |||
Some lavas of unusual composition have erupted onto the surface of the Earth. These include: | |||
* ] and ] lavas are known from ] volcano in ], which is the sole example of an active carbonatite volcano.<ref> {{Webarchive|url=https://web.archive.org/web/20171023185741/http://www.geology.sdsu.edu/how_volcanoes_work/Unusual%20lava.html |date=2017-10-23 }}, ], Geology</ref> Carbonatites in the geologic record are typically 75% carbonate minerals, with lesser amounts of silica-undersaturated silicate minerals (such as ]s and olivine), ], ], and ]. This may not reflect the original composition of the lava, which may have included ] that was subsequently removed by hydrothermal activity, though laboratory experiments show that a calcite-rich magma is possible. Carbonatite lavas show ]s indicating they are derived from the highly alkaline silicic lavas with which they are always associated, probably by separation of an immiscible phase.{{sfn|Philpotts|Ague|2009|pp=396-397}} Natrocarbonatite lavas of Ol Doinyo Lengai are composed mostly of sodium carbonate, with about half as much calcium carbonate and half again as much potassium carbonate, and minor amounts of halides, fluorides, and sulphates. The lavas are extremely fluid, with viscosities only slightly greater than water, and are very cool, with measured temperatures of {{cvt|491|to|544|C}}.<ref>{{cite journal |last1=Keller |first1=Jörg |last2=Krafft |first2=Maurice |title=Effusive natrocarbonatite activity of Oldoinyo Lengai, June 1988 |journal=Bulletin of Volcanology |date=November 1990 |volume=52 |issue=8 |pages=629–645 |doi=10.1007/BF00301213|bibcode=1990BVol...52..629K |s2cid=129106033 }}</ref> | |||
* ] lavas are thought to be the source of the ] at ], ] which formed during the ].<ref name="IronOxidelava"/> Iron oxide lavas of ] age occur at the ] volcanic complex on the Chile-Argentina border.<ref name="ChileIronOxideLava"/> Iron oxide lavas are thought to be the result of ] separation of iron oxide magma from a parental magma of ] or alkaline composition.<ref name="IronOxidelava"/> | |||
* ] lava flows up to {{convert|250|m|0|abbr=off}} long and {{convert|10|m|0|abbr=off}} wide occur at ] volcano, Chile. They were formed by the melting of sulfur deposits at temperatures as low as {{convert|113|°C}}.<ref name="ChileIronOxideLava"/> | |||
The term "lava" can also be used to refer to molten "ice mixtures" in eruptions on the icy ] of the ]'s ]s.<ref>{{cite book|editor-last1=McBride|editor-last2=Gilmore|year=2007|title=An introduction to the Solar System|publisher=]|page=392}}</ref> | |||
===Sheeted flows=== | |||
Sheeted flows are an uncommon form of eruptive phenomena of felsic and intermediate volcanoes. Internal pressure of gases tend to promote pyroclastic and explosive eruptions. However, a viscous magma will flow, though very slowly, across the surface of the Earth. | |||
=== Rheology === | |||
Typically the lava flow forms a ''sheeted flow'' or ''laminar flow'', with the upper and lower margins of the flowing lava forming a hard, brittle shell inside of which the sticky, viscous lava will be flowing. The hard skin forms a chaotic igneous ] called ''autobreccia'', as the flow creeps along, churning the outer margins apart. This is similar to an a’a flow except that the inner lava will show evidence of stretching, plastic deformation and even ] of the highly viscous lava. | |||
] on the east rift zone of ] Volcano in ]]] | |||
The lava's viscosity mostly determines the behavior of lava flows. While the temperature of common silicate lava ranges from about {{cvt|800|C}} for felsic lavas to {{cvt|1200|C}} for mafic lavas,{{sfn|Philpotts|Ague|2009|p=20}} its viscosity ranges over seven orders of magnitude, from 10<sup>11</sup> cP (10<sup>8</sup> Pa⋅s) for felsic lavas to 10<sup>4</sup> cP (10 Pa⋅s) for mafic lavas.{{sfn|Philpotts|Ague|2009|p=20}} Lava viscosity is mostly determined by composition but also depends on temperature{{sfn|Philpotts|Ague|2009|p=23}} and shear rate.<ref name=Sonder>{{cite journal|last1=Sonder|first1=I|last2=Zimanowski|first2=B|last3=Büttner|first3=R|year=2006|title=Non-Newtonian viscosity of basaltic magma|journal=Geophysical Research Letters|volume=330|issue=2|pages=L02303|doi=10.1029/2005GL024240|bibcode=2006GeoRL..33.2303S|doi-access=free}}</ref> | |||
Lava viscosity determines the ] that takes place when the lava is erupted. The greater the viscosity, the greater the tendency for eruptions to be explosive rather than effusive. As a result, most lava flows on Earth, Mars, and Venus are composed of basalt lava.{{sfn|Schmincke|2003|p=128}} On Earth, 90% of lava flows are mafic or ultramafic, with intermediate lava making up 8% of flows and felsic lava making up just 2% of flows.<ref name="umass">{{cite web |title=Lava Flows |url=http://www.geo.umass.edu/courses/volcanology/Lava%20Flows.pdf |website=UMass Department of Geosciences |publisher=University of Massachusetts Amherst |access-date=5 June 2018 |pages=19 |date=11 February 2004}}</ref> Viscosity also determines the aspect (thickness relative to lateral extent) of flows, the speed with which flows move, and the surface character of the flows.{{sfn|Philpotts|Ague|2009|p=23}}<ref name="PetersonTilling1980">{{cite journal |last1=Peterson |first1=Donald W. |last2=Tilling |first2=Robert I. |title=Transition of basaltic lava from pahoehoe to aa, Kilauea Volcano, Hawaii: Field observations and key factors |journal=Journal of Volcanology and Geothermal Research |date=May 1980 |volume=7 |issue=3–4 |pages=271–293 |doi=10.1016/0377-0273(80)90033-5|bibcode=1980JVGR....7..271P }}</ref> | |||
Examples of laminar or sheeted flows include the ] aged volcanic edifices of the ], and the cliffs of ] in Brisbane, Australia. | |||
When highly viscous lavas erupt effusively rather than in their more common explosive form, they almost always erupt as high-aspect flows or domes. These flows take the form of block lava rather than {{okina}}a{{okina}}ā or pāhoehoe. Obsidian flows are common.{{sfn|Schmincke|2003|pp=132-138}} Intermediate lavas tend to form steep stratovolcanoes, with alternating beds of lava from effusive eruptions and tephra from explosive eruptions.{{sfn|Schmincke|2003|pp=143-144}} Mafic lavas form relatively thin flows that can move great distances, forming shield volcanoes with gentle slopes.{{sfn|Schmincke|2003|pp=127-128}} | |||
===‘A‘a=== | |||
] in ].]] | |||
In addition to melted rock, most lavas contain solid crystals of various minerals, fragments of exotic rocks known as ]s, and fragments of previously solidified lava. The crystal content of most lavas gives them ] and ] properties.<ref>{{cite journal |title=Transient phenomena in vesicular lava flows based on laboratory experiments with analogue materials |journal=Journal of Volcanology and Geothermal Research|volume=132 |issue=2–3 |pages=115–136 |doi=10.1016/s0377-0273(03)00341-x |last1=Pinkerton |first1=H. |first2=N. |last2=Bagdassarov |year=2004 |bibcode=2004JVGR..132..115B}}</ref> In other words, most lavas do not behave like Newtonian fluids, in which the rate of flow is proportional to the ]. Instead, a typical lava is a ], which shows considerable resistance to flow until a stress threshold, called the yield stress, is crossed.{{sfn|Schmincke|2003|pp=39-40}} This results in ] of partially crystalline lava. A familiar example of plug flow is toothpaste squeezed out of a toothpaste tube. The toothpaste comes out as a semisolid plug, because shear is concentrated in a thin layer in the toothpaste next to the tube and only there does the toothpaste behave as a fluid. Thixotropic behavior also hinders crystals from settling out of the lava.{{sfn|Philpotts|Ague|2009|p=40}} Once the crystal content reaches about 60%, the lava ceases to behave like a fluid and begins to behave like a solid. Such a mixture of crystals with melted rock is sometimes described as ''crystal mush''.{{sfn|Philpotts|Ague|2009|p=16}} | |||
'''Aa''' or '''‘A‘a''' (], from ]: ''‘a‘ā'' meaning "stoney with rough lava", but also to "burn" or "blaze") is one of three basic types of flow lava. ‘A‘a is characterized by a rough or rubbly surface composed of broken lava blocks called ]. | |||
Lava flow speeds vary based primarily on viscosity and slope. In general, lava flows slowly, with typical speeds for Hawaiian basaltic flows of {{cvt|0.25|mph|sigfig=2|order=flip}} and maximum speeds of {{cvt|6|to|30|mph|0|order=flip}} on steep slopes.<ref name=umass/> An exceptional speed of {{cvt|20|to|60|mph|order=flip}} was recorded following the collapse of a lava lake at ].<ref name=umass/> The scaling relationship for lavas is that the average speed of a flow scales as the square of its thickness divided by its viscosity.{{sfn|Philpotts|Ague|2009|p=71}} This implies that a rhyolite flow would have to be about a thousand times thicker than a basalt flow to flow at a similar speed. | |||
The loose, broken, and sharp, spiny surface of a solidified ‘a‘a flow makes walking difficult and slow. The clinkery surface actually covers a massive dense core, which was the most active part of the flow. As pasty lava in the core travels downslope, the clinkers are carried along at the surface. At the leading edge of an ‘a‘a flow, however, these cooled fragments tumble down the steep front and are buried by the advancing flow. This produces a layer of lava fragments both at the bottom and top of an ‘a‘a flow. Accretionary lava balls as large as 3 m (10 ft) are common on ‘a‘a flows. ‘A‘a is usually of higher viscosity than pāhoehoe (usually spelled just pahoehoe). Pāhoehoe can turn into ‘a‘a if it becomes turbulent due to meeting impediments or steep slopes. | |||
=== |
===Temperature=== | ||
] in ]]] | |||
] on the east rift zone of ] Volcano in ].]] | |||
The temperature of most types of molten lava ranges from about {{cvt|800|C}} to {{cvt|1200|C}} {{sfn|Philpotts|Ague|2009|p=20}} depending on the lava's chemical composition. This temperature range is similar to the hottest temperatures achievable with a forced air charcoal forge.<ref>{{cite journal |last1=Cheng |first1=Zhilong |last2=Yang |first2=Jian |last3=Zhou |first3=Lang |last4=Liu |first4=Yan |last5=Wang |first5=Qiuwang |title=Characteristics of charcoal combustion and its effects on iron-ore sintering performance |journal=Applied Energy |date=January 2016 |volume=161 |pages=364–374 |doi=10.1016/j.apenergy.2015.09.095|bibcode=2016ApEn..161..364C }}</ref> Lava is most fluid when first erupted, becoming much more viscous as its temperature drops.{{sfn|Philpotts|Ague|2009|p=23}} | |||
'''Pahoehoe''' or '''Pāhoehoe''' (Hawaiian English, from Hawaiian, meaning "smooth, unbroken lava") is ]ic ] that has a smooth, hummocky, or ropy surface. A pāhoehoe flow typically advances as a series of small lobes and toes that continually break out from a cooled crust. The surface texture of pāhoehoe flows varies widely, displaying all kinds of bizarre shapes often referred to as lava sculpture. | |||
Lava flows quickly develop an insulating crust of solid rock as a result of radiative loss of heat. Thereafter, the lava cools by a very slow conduction of heat through the rocky crust. For instance, geologists of the ] regularly drilled into the Kilauea Iki lava lake, formed in an eruption in 1959. After three years, the solid surface crust, whose base was at a temperature of {{cvt|1065|C}}, was still only {{cvt|14|m}} thick, even though the lake was about {{cvt|100|m}} deep. Residual liquid was still present at depths of around {{cvt|80|m}} nineteen years after the eruption.{{sfn|Philpotts|Ague|2009|p=20}} | |||
=== Pillow lava === | |||
A cooling lava flow shrinks, and this fractures the flow. Basalt flows show a characteristic pattern of fractures. The uppermost parts of the flow show irregular downward-splaying fractures, while the lower part of the flow shows a very regular pattern of fractures that break the flow into five- or six-sided columns. The irregular upper part of the solidified flow is called the ''entablature'', while the lower part that shows ] is called the ''colonnade''. (The terms are borrowed from Greek temple architecture.) Likewise, regular vertical patterns on the sides of columns, produced by cooling with periodic fracturing, are described as ''chisel marks''. Despite their names, these are natural features produced by cooling, thermal contraction, and fracturing.{{sfn|Philpotts|Ague|2009|pp=55-56}} | |||
] | |||
'''Pillow lava''' is the rock type typically formed when lava emerges from an ] ] or a lava flow enters the ocean. The viscous lava gains a solid crust immediately upon contact with the water, and this crust cracks and oozes additional large blobs or "pillows" as more lava emerges from the advancing flow. Since the majority of ]'s surface is covered by water, and most volcanoes are situated near or under it, pillow lava is actually very common. | |||
As lava cools, crystallizing inwards from its edges, it expels gases to form vesicles at the lower and upper boundaries. These are described as ''pipe-stem vesicles'' or ''pipe-stem amygdales''. Liquids expelled from the cooling crystal mush rise upwards into the still-fluid center of the cooling flow and produce vertical ''vesicle cylinders''. Where these merge towards the top of the flow, they form sheets of vesicular basalt and are sometimes capped with gas cavities that sometimes fill with secondary minerals. The beautiful ] geodes found in the flood basalts of South America formed in this manner.{{sfn|Philpotts|Ague|2009|pp=58-59}} | |||
== Lava landforms == | |||
Due to being formed from viscous molten rock, lava flows and eruptions create distinctive formations, landforms and topographical features from the macroscopic to the microscopic. | |||
Flood basalts typically crystallize little before they cease flowing, and, as a result, flow textures are uncommon in less silicic flows.{{sfn|Philpotts|Ague|2009|p=48}} On the other hand, ] is common in felsic flows.{{sfn|Philpotts|Ague|2009|p=72}} | |||
There are few things not made of rock that can resist the progress of flowing lava; trees, houses, everything yields to its massive assault. Trees soon catch fire due to the heat of approaching lava, and when the lava reaches them they emit a hissing noise, almost amounting to a shriek. The trees then plunge into the | |||
molten flood and are seen no more. Even the sea cannot stop the lava-stream, but retires on its approach; promontories stretching a considerable distance from the shore are formed in this manner as the molten lava hardens into stone. | |||
==Lava morphology== | |||
], ]]] | |||
The morphology of lava describes its surface form or texture. More fluid basaltic lava flows tend to form flat sheet-like bodies, whereas viscous rhyolite lava flows form knobbly, blocky masses of rock. Lava erupted underwater has its own distinctive characteristics. | |||
] at ]. ]] | |||
=== {{okina}}A{{okina}}ā === | |||
{{hatnote|The following subsection is about the types of lava flow. For the sculpture of a god from the Pacific island of Rurutu, see ].}} | |||
] in ], United States]] | |||
''{{okina}}A{{okina}}ā'' (also spelled ''aa'', ''a{{okina}}a'', ''{{okina}}a{{okina}}a'', and ''a-aa'', and pronounced {{IPA|haw|ʔəˈʔaː|}} or {{IPAc-en|ˈ|ɑː|(|ʔ|)|ɑː}}) is one of three basic types of flow lava. {{okina}}A{{okina}}ā is basaltic lava characterized by a rough or rubbly surface composed of broken lava blocks called clinker. The word is ] meaning "stony rough lava", but also to "burn" or "blaze";<ref>{{cite dictionary|title={{okina}}a{{okina}}ā|url=http://www.wehewehe.org/gsdl2.5/cgi-bin/hdict?d=D10|dictionary=Hawaiian Dictionary (Hwn to Eng)|archive-url=https://archive.today/20121228131017/http://www.wehewehe.org/gsdl2.5/cgi-bin/hdict?d=D10 |archive-date=28 December 2012}}</ref> it was introduced as a technical term in geology by ].<ref name="Kemp">{{cite book |first1=James Furman |last1=Kemp |title=A handbook of rocks for use without the microscope : with a glossary of the names of rocks and other lithological terms |volume=5 |location=New York |publisher=D. Van Nostrand |year=1918 |pages=180, 240 |url=https://books.google.com/books?id=tHQNAAAAYAAJ&pg=PA180}}</ref><ref name="dutton">{{cite journal |page=240 |first1=C. E. |last1=Dutton |journal=Annual Report U.S. Geological Survey |volume=4 |title=Hawaiian volcanoes |year=1883 |number=95}}</ref> | |||
The loose, broken, and sharp, spiny surface of an {{okina}}a{{okina}}ā flow makes ] difficult and slow. The clinkery surface actually covers a massive dense core, which is the most active part of the flow. As pasty lava in the core travels downslope, the clinkers are carried along at the surface. At the leading edge of an {{okina}}a{{okina}}ā flow, however, these cooled fragments tumble down the steep front and are buried by the advancing flow. This produces a layer of lava fragments both at the bottom and top of an {{okina}}a{{okina}}ā flow.{{sfn|Schmincke|2003|pp=131-132}} | |||
Accretionary lava balls as large as {{convert|3|m|0|abbr=off}} are common on {{okina}}a{{okina}}ā flows.<ref>{{cite book |last1=Macdonald |first1=Gordon A. |last2=Abbott |first2=Agatin T. |last3=Peterson |first3=Frank L. |title=Volcanoes in the sea : the geology of Hawaii |date=1983 |publisher=University of Hawaii Press |location=Honolulu |isbn=0824808320 |page=23 |edition=2nd}}</ref> {{okina}}A{{okina}}ā is usually of higher viscosity than pāhoehoe. Pāhoehoe can turn into {{okina}}a{{okina}}ā if it becomes turbulent from meeting impediments or steep slopes.{{sfn|Schmincke|2003|pp=131-132}} | |||
The sharp, angled texture makes {{okina}}a{{okina}}ā a strong ] reflector, and can easily be seen from an orbiting satellite (bright on ] pictures).<ref name="VenusAa">{{cite web | url=http://www.lpi.usra.edu/publications/slidesets/hawaii/ | title=Radar Studies of Lava Flows | publisher=Lunar and Planetary Institute | work=Volcanic Features of Hawaii and Other Worlds | access-date=18 March 2017 | author=McGounis-Mark, Peter}}</ref> | |||
{{okina}}A{{okina}}ā lavas typically erupt at temperatures of {{convert|1050|to|1150|°C}} or greater.<ref>{{cite journal |last1=Pinkerton |first1=Harry |last2=James |first2=Mike |last3=Jones |first3=Alun |title=Surface temperature measurements of active lava flows on Kilauea volcano, Hawai'i |journal=Journal of Volcanology and Geothermal Research |date=March 2002 |volume=113 |issue=1–2 |pages=159–176 |doi=10.1016/S0377-0273(01)00257-8|bibcode=2002JVGR..113..159P }}</ref><ref>{{cite journal |last1=Cigolini |first1=Corrado |last2=Borgia |first2=Andrea |last3=Casertano |first3=Lorenzo |title=Intra-crater activity, aa-block lava, viscosity and flow dynamics: Arenal Volcano, Costa Rica |journal=Journal of Volcanology and Geothermal Research |date=March 1984 |volume=20 |issue=1–2 |pages=155–176 |doi=10.1016/0377-0273(84)90072-6|bibcode=1984JVGR...20..155C }}</ref> | |||
===Pāhoehoe=== | |||
] | |||
''Pāhoehoe'' (also spelled ''pahoehoe'', from Hawaiian {{IPA|haw|paːˈhoweˈhowe|}}<ref>{{cite dictionary|title=pāhoehoe|url=http://www.wehewehe.org/gsdl2.5/cgi-bin/hdict?d=D16177|dictionary=Hawaiian Dictionary (Hwn to Eng)|archive-url=https://archive.today/20120918075604/http://www.wehewehe.org/gsdl2.5/cgi-bin/hdict?d=D16177 |archive-date=18 September 2012}}</ref> meaning "smooth, unbroken lava") is basaltic lava that has a smooth, billowy, undulating, or ropy surface. These surface features are due to the movement of very fluid lava under a congealing surface crust. The Hawaiian word was introduced as a technical term in geology by ].<ref name="Kemp" /><ref name=dutton/> | |||
A pāhoehoe flow typically advances as a series of small lobes and toes that continually break out from a cooled crust. It also forms ]s where the minimal heat loss maintains a low viscosity. The surface texture of pāhoehoe flows varies widely, displaying all kinds of bizarre shapes often referred to as lava sculpture. With increasing distance from the source, pāhoehoe flows may change into {{okina}}a{{okina}}ā flows in response to heat loss and consequent increase in viscosity.{{sfn|Schmincke|2003|pp=128-132}} Experiments suggest that the transition takes place at a temperature between {{convert|1200 and 1170|C}}, with some dependence on shear rate.<ref>{{cite journal |last1=Sehlke |first1=A. |last2=Whittington |first2=A. |last3=Robert |first3=B. |last4=Harris |first4=A. |last5=Gurioli |first5=L. |last6=Médard |first6=E. |title=Pahoehoe to 'a'a transition of Hawaiian lavas: an experimental study |journal=Bulletin of Volcanology |date=17 October 2014 |volume=76 |issue=11 |pages=876 |doi=10.1007/s00445-014-0876-9|s2cid=129019507 }}</ref><ref name=Sonder/> Pahoehoe lavas typically have a temperature of {{convert|1100|to|1200|°C}}.{{sfn|Philpotts|Ague|2009|p=20}} | |||
On the Earth, most lava flows are less than {{convert|10|km|mi|abbr=on}} long, but some pāhoehoe flows are more than {{convert|50|km|mi|abbr=on}} long.<ref name="LavaLength">{{cite web | url=http://volcano.si.edu/learn_galleries.cfm?p=12 | title=Types and Processes Gallery: Lava Flows | publisher=] | work=Global Volcanism Program | date=2013 | access-date=1 December 2015}}</ref> Some flood basalt flows in the geologic record extend for hundreds of kilometres.{{sfn|Philpotts|Ague|2009|p=53}} | |||
The rounded texture makes pāhoehoe a poor radar reflector, and is difficult to see from an orbiting satellite (dark on Magellan picture).<ref name="VenusAa"/> | |||
=== Block lava flows === | |||
] in Lassen Volcanic National Park]] | |||
Block lava flows are typical of andesitic lavas from stratovolcanoes. They behave in a similar manner to {{okina}}a{{okina}}ā flows but their more viscous nature causes the surface to be covered in smooth-sided angular fragments (blocks) of solidified lava instead of clinkers. As with {{okina}}a{{okina}}ā flows, the molten interior of the flow, which is kept insulated by the solidified blocky surface, advances over the rubble that falls off the flow front. They also move much more slowly downhill and are thicker in depth than {{okina}}a{{okina}}ā flows. | |||
{{sfn|Schmincke|2003|p=132}} | |||
===Pillow lava===<!-- This section is linked from ] --> | |||
{{Main|Pillow lava}} | |||
] on the ocean floor near Hawaii]] | |||
''Pillow lava'' is the lava structure typically formed when lava emerges from an ] or ] or a lava flow enters the ocean. The viscous lava gains a solid crust on contact with the water, and this crust cracks and oozes additional large blobs or "pillows" as more lava emerges from the advancing flow. Since water covers the majority of ]'s surface and most volcanoes are situated near or under bodies of water, pillow lava is very common.<ref>{{cite journal |journal=Bulletin of the Geological Society of America |last1=Lewis |first1=J.V. |title=Origin of pillow lavas |volume=25 |year=1914 |issue=1 |page=639 |doi=10.1130/GSAB-25-591 |bibcode=1914GSAB...25..591L |url=https://books.google.com/books?id=ItaPAAAAMAAJ&pg=PA639}}</ref> | |||
== Lava landforms == | |||
Because it is formed from viscous molten rock, lava flows and eruptions create distinctive formations, landforms and topographical features from the macroscopic to the microscopic. | |||
===Volcanoes=== | ===Volcanoes=== | ||
{{Main|Volcano}} | |||
] is a composite volcanic cone formed from basaltic andesite.]]]es are the primary landform created by lava eruption and consist of flattish, shallow shield volcanes formed from basalt to steeply-sided ash and lava composite volcanic cones typical of andesite an rhyolite lavas. | |||
], Costa Rica, is a ].]] | |||
Volcanoes are the primary landforms built by repeated eruptions of lava and ash over time. They range in shape from ]es with broad, shallow slopes formed from predominantly effusive eruptions of relatively fluid basaltic lava flows, to steeply-sided ]es (also known as composite volcanoes) made of alternating layers of ash and more viscous lava flows typical of intermediate and felsic lavas.{{sfn|Philpotts|Ague|2009|pp=59-73}} | |||
A ], which is a large subsidence crater, can form in a stratovolcano, if the magma chamber is partially or wholly emptied by large explosive eruptions; the summit cone no longer supports itself and thus collapses in on itself afterwards.{{sfn|Schmincke|2003|pp=147-148}} Such features may include volcanic crater lakes and lava domes after the event.{{sfn|Schmincke|2003|pp=132, 286}} However, calderas can also form by non-explosive means such as gradual magma subsidence. This is typical of many shield volcanoes.{{sfn|Schmincke|2003|pp=149-151}} | |||
Volcanoes can form ]s if they are obliterated by large phyoclastic or ] eruptions, and such features typically include volcanic crater lakes and lava domes after the event. | |||
===Cinder and |
===Cinder and spatter cones=== | ||
{{Main|Volcanic cone}} | |||
Cinder cones and ''spatter cones'' are small-scale features formed by lava accumulation around a small vent on a volcanic edifice. Cinder cones are formed from ] or ] and ] which is thrown from an explosive vent. Spatter cones are formed by accumulation of molten volcanic slag and cinders ejected in a more liquid form. | |||
]s and spatter cones are small-scale features formed by lava accumulation around a small vent on a volcanic edifice. Cinder cones are formed from ] or ] and ] which is thrown from an explosive vent. Spatter cones are formed by accumulation of molten volcanic slag and cinders ejected in a more liquid form.{{sfn|Macdonald|Abbott|Peterson|1983|pp=26-17}} | |||
=== |
===Kīpukas=== | ||
{{Main|Kīpuka}} | |||
], the largest meadow in the Valles Caldera National Preserve.]] | |||
Another ] term derived from the ], a kīpuka denotes an elevated area such as a hill, ridge or old lava dome inside or downslope from an area of active volcanism. New lava flows will cover the surrounding land, isolating the kīpuka so that it appears as a (usually) forested island in a barren lava flow.{{sfn|Macdonald|Abbott|Peterson|1983|pp=22-23}} | |||
]s are formed by the extrusion of viscous felsic magma. They can form prominent rounded protuberances, such as at ]. | |||
===Lava |
===Lava domes and coulées=== | ||
{{Main|Lava dome}} | |||
Lava tubes are formed when a flow of relatively fluid lava cools on the upper surface sufficiently to form a crust. Beneath this crust, which by dint of being made of rock is an excellent insulator, the lava can continue to flow as a liquid. When this flow occurs over a prolonged period of time the lava conduit can form a tunnel-like apertre or '']'', which can conduct molten rock many kilometres from the vent without cooling appreciably. Often these lava tubes drain out once the suppply of fresh lava has stopped, leaving a considerable length of open tunnel within the lava flow. | |||
], New Mexico, United States]] | |||
Lava domes are formed by the extrusion of viscous felsic magma. They can form prominent rounded protuberances, such as at ]. As a volcano extrudes silicic lava, it can form an ''inflation dome'' or ''endogenous dome'', gradually building up a large, pillow-like structure which cracks, fissures, and may release cooled chunks of rock and rubble. The top and side margins of an inflating lava dome tend to be covered in fragments of rock, ] and ash.{{sfn|Schmincke|2003|pp=132-138, 152-153}} | |||
Examples of lava dome eruptions include the ] dome, and successive lava domes of ].{{sfn|Schmincke|2003|pp=132-134}} | |||
Lava tubes are known from the modern day eruptions of Kilauhea, and significant, extensive lava tubes of Tertiary age are known from North ], ], some extending for 15 kilometres. | |||
When a dome forms on an inclined surface it can flow in short thick flows called coulées (dome flows). These flows often travel only a few kilometres from the vent.{{sfn|Schmincke|2003|pp=132-138}} | |||
=== Lava cascades and fountains === | |||
] | |||
===Lava tubes=== | |||
The eruptions of lava are sometimes attended by peculiarities which impart to them much additional grandeur. Instances have occurred in which the fiery stream has plunged over a sheer precipice of immense height, so as to produce a glowing cascade exceeding (in breadth and perpendicular descent) the celebrated ]. In other cases, the lava, instead of at once flowing down the sides of the mountain, has been first thrown up into the air as a fiery fountain several hundred feet in height (see ]). | |||
{{Main|Lava tube}} | |||
Lava tubes are formed when a flow of relatively fluid lava cools on the upper surface sufficiently to form a crust. Beneath this crust, which being made of rock is an excellent insulator, the lava can continue to flow as a liquid. When this flow occurs over a prolonged period of time the lava conduit can form a tunnel-like aperture or ''lava tube'', which can conduct molten rock many kilometres from the vent without cooling appreciably. Often these lava tubes drain out once the supply of fresh lava has stopped, leaving a considerable length of open tunnel within the lava flow.{{sfn|Macdonald|Abbott|Peterson|1983|pp=23,26-29}} | |||
Lava tubes are known from the modern day eruptions of Kīlauea,{{sfn|Macdonald|Abbott|Peterson|1983|p=27}} and significant, extensive and open lava tubes of Tertiary age are known from North ], ], some extending for {{convert|15|km|0|abbr=off}}.<ref>{{cite journal |last1=Atkinson |first1=A. |last2=Griffin |first2=T. J. |last3=Stephenson |first3=P. J. |title=A major lava tube system from Undara Volcano, North Queensland |journal=Bulletin Volcanologique |date=June 1975 |volume=39 |issue=2 |pages=266–293 |doi=10.1007/BF02597832|bibcode=1975BVol...39..266A |s2cid=129126355 }}</ref> | |||
===Lava lakes=== | ===Lava lakes=== | ||
{{Main|Lava lake}} | |||
Rarely, a volcanic cone may fill with lava but not erupt. Lava which pools wihin the caldera is known as a lava lake. Lava lakes do not usually persist for long, either draining back into the magma chamber once pressure is relieved (usually by venting of gases through the caldera), or by draining via eruption of lava flows or pyroclastic explosion. | |||
], New Mexico, United States: a ] in the distance, with a radiating ] on its south side]] | |||
Rarely, a volcanic cone may fill with lava but not erupt. Lava which pools within the caldera is known as a lava lake.{{sfn|Schmincke|2003|p=27}} Lava lakes do not usually persist for long, either draining back into the magma chamber once pressure is relieved (usually by venting of gases through the caldera), or by draining via eruption of lava flows or pyroclastic explosion. | |||
There are only a few sites in the world where permanent lakes of lava exist. These include: | There are only a few sites in the world where permanent lakes of lava exist. These include: | ||
* ], ]<ref name="lava-lake">{{cite journal |last1=Lev |first1=Einat |last2=Ruprecht |first2=Philipp |last3=Oppenheimer |first3=Clive |last4=Peters |first4=Nial |last5=Patrick |first5=Matt |last6=Hernández |first6=Pedro A. |last7=Spampinato |first7=Letizia |last8=Marlow |first8=Jeff |title=A global synthesis of lava lake dynamics |journal=Journal of Volcanology and Geothermal Research |date=September 2019 |volume=381 |pages=16–31 |doi=10.1016/j.jvolgeores.2019.04.010|bibcode=2019JVGR..381...16L |s2cid=182844266 |doi-access=free }}</ref> | |||
*], ] | |||
* ], ]{{sfn|Philpotts|Ague|2009|p=61}} | |||
*], ] | |||
* ], ]<ref>{{cite journal |last1=Burgi |first1=P.-Y. |last2=Darrah |first2=T. H. |last3=Tedesco |first3=D. |last4=Eymold |first4=W. K. |title=Dynamics of the Mount Nyiragongo lava lake: DYNAMICS OF THE MT. NYIRAGONGO LAVA LAKE |journal=Journal of Geophysical Research: Solid Earth |date=May 2014 |volume=119 |issue=5 |pages=4106–4122 |doi=10.1002/2013JB010895|doi-access=free }}</ref> | |||
*], ] | |||
*], ] | * ], ].<ref name="lava-lake"/> | ||
===Lava delta=== | |||
== Composition of volcanic rocks == | |||
{{Main|Lava delta}} | |||
] | |||
Lava deltas form wherever ] flows of lava enter standing bodies of water. The lava cools and breaks up as it encounters the water, with the resulting fragments filling in the ] such that the sub-aerial flow can move further offshore. Lava deltas are generally associated with large-scale, effusive type basaltic volcanism.<ref>{{cite journal |last1=Bosman |first1=Alessandro |last2=Casalbore |first2=Daniele |last3=Romagnoli |first3=Claudia |last4=Chiocci |first4=Francesco Latino |title=Formation of an 'a'ā lava delta: insights from time-lapse multibeam bathymetry and direct observations during the Stromboli 2007 eruption |journal=Bulletin of Volcanology |date=July 2014 |volume=76 |issue=7 |pages=838 |doi=10.1007/s00445-014-0838-2|bibcode=2014BVol...76..838B |s2cid=129797425 }}</ref> | |||
== Lava fountains == | |||
The sub-family of rocks which form from volcanic lava are called ]s (to differentiate them from igneous rocks which form from magma, below the surface of the earth, called ]s). | |||
]]] | |||
A '''lava fountain''' is a ] phenomenon in which lava is forcefully but non-explosively ejected from a ], vent, or ]. The highest lava fountain recorded was during the 23 November 2013 eruption of ] in Italy, which reached a stable height of around {{convert|2500|m|ft|sigfig=2|abbr=on}} for 18 minutes, briefly peaking at a height of {{convert|3400|m|ft|sigfig=2|abbr=on}}.<ref>{{cite journal |last1=Bonaccorso |first1=A. |last2=Calvari |first2=S. |last3=Linde |first3=A. |last4=Sacks |first4=S. |title=Eruptive processes leading to the most explosive lava fountain at Etna volcano: The 23 November 2013 episode |journal=Geophysical Research Letters |date=28 July 2014 |volume=41 |issue=14 |pages=4912–4919 |doi=10.1002/2014GL060623 |bibcode=2014GeoRL..41.4912B |s2cid=129813334 |quote=To the best of our knowledge, it reached the highest value ever measured for a lava fountain on Earth.}}</ref> Lava fountains may occur as a series of short pulses, or a continuous jet of lava. They are commonly associated with ]s.{{sfn|Macdonald|Abbott|Peterson|1983|p=9}} | |||
The lavas of different volcanoes, when cooled and hardened, differ much in their appearance and composition. If a ] lava-stream cools quickly, it can quickly freeze into a black glassy substance called ]. When filled with bubbles of gas, the same lava may form the spongy mineral ]. Allowed to cool slowly, it forms a light-colored, uniformly solid rock called ]. | |||
==Hazards== | |||
==Towns destroyed by lava== | |||
Lava flows are enormously destructive to property in their path. However, casualties are rare since flows are usually slow enough for people and animals to escape, though this is dependent on the viscosity of the lava. Nevertheless, injuries and deaths have occurred, either because they had their escape route cut off, because they got too close to the flow<ref name=usgs> USGS</ref> or, more rarely, if the lava flow front travels too quickly. This notably happened during the eruption of ] in Zaire (now ]). On the night of 10 January 1977, a crater wall was breached and a fluid lava lake drained out in under an hour. The resulting flow sped down the steep slopes at up to {{convert|100|km/h|abbr=on}}, and overwhelmed several villages while residents were asleep. As a result of this disaster, the mountain was designated a ] in 1991.<ref name=hvo> ] ]</ref> | |||
] in 1990.]] | |||
] frequently have a different cause. For example, volcanic ejecta, ] from a collapsing lava dome, ]s, poisonous gases that travel ahead of lava, or explosions caused when the flow comes into contact with water.<ref name=usgs /> A particularly dangerous area is called a ]. This very young ground will typically break off and fall into the sea. | |||
*] (abandoned) | |||
*] (abandoned) | |||
*] (abandoned) | |||
*] (abandoned) | |||
*] (abandoned) | |||
*] (rebuilt) | |||
Areas of recent lava flows continue to represent a hazard long after the lava has cooled. Where young flows have created new lands, land is more unstable and can break off into the sea. Flows often crack deeply, forming dangerous chasms, and a fall against {{okina}}a{{okina}}ā lava is similar to falling against broken glass. Rugged hiking boots, long ], and gloves are recommended when crossing lava flows. | |||
==Towns partially destroyed by lava flows== | |||
*], in the eruption ] in ] (rebuilt) | |||
Diverting a lava flow is extremely difficult, but it can be accomplished in some circumstances, as was once partially achieved in ], Iceland.<ref>{{cite web |url=http://indianapublicmedia.org/amomentofscience/vestmannaeyjar-town-fought-volcano-won/ |title=Vestmannaeyjar, The Town That Fought A Volcano And Won |last1=Sonstroem |first1=Eric |date=14 September 2010 |website=indianapublicmedia.org |publisher=Indiana Public Media |access-date=24 November 2017 |archive-date=23 February 2017 |archive-url=https://web.archive.org/web/20170223203301/http://indianapublicmedia.org/amomentofscience/vestmannaeyjar-town-fought-volcano-won/ |url-status=dead }}</ref> The optimal design of simple, low-cost barriers that divert lava flows is an area of ongoing research.<ref>{{cite journal|url=https://www.nature.com/articles/ngeo2470|title=Diverting lava flows in the lab|last1=Dietterich |first1=Hannah|last2=Cashman |first2=Katherine|last3=Rust |first3=Alison |last4=Lev |first4=Einat |date=2015 |journal=Nature Geoscience |volume=8|issue=7|pages=494–496|doi=10.1038/ngeo2470|bibcode=2015NatGe...8..494D}}</ref><ref>{{cite journal|url=https://doi.org/10.1103/PhysRevFluids.5.084101|title=Viscous free-surface flows past cylinders|last1=Hinton |first1=Edward|last2=Hogg |first2=Andrew|last3=Huppert |first3=Herbert |date=2020 |journal=Physical Review Fluids |volume=5|number=84101|page=084101|doi=10.1103/PhysRevFluids.5.084101|bibcode=2020PhRvF...5h4101H|hdl=1983/f52f7078-5936-4e37-9d79-be456f08eb5c |s2cid=225416948|hdl-access=free}}</ref> | |||
*], in the eruption of ] in ] | |||
*], in the ] ] eruption (rebuilt) | |||
===Towns destroyed by lava flows=== | |||
*], by the eruption of ] in ]-] (abandoned) | |||
], United States, in 1990.]] | |||
*], Italy (buried) | |||
* The ] villages of ] and Wii Lax K'abit in northwestern ], Canada, were destroyed by thick lava flows during the eruption of ] in the 1700s. | |||
*], Italy (buried beneath modern ]) | |||
* ] on the island of ] was destroyed by the ] (rebuilt). | |||
*] (village the volcano was named after) and ], Mexico, by ] from 1943-1952. | |||
* ], ], buried by lava erupted from ] in 1814<ref>{{cite web |url=http://www.nscb.gov.ph/Ru5/overview/albay/attractions.html |title=Tourist attractions of Albay Province, Philippines |publisher=Nscb.gov.ph |access-date=2013-12-08 |url-status=dead |archive-url=https://web.archive.org/web/20160921155807/http://www.nscb.gov.ph/ru5/overview/albay/attractions.html |archive-date=2016-09-21 }}</ref> | |||
* Keawaiki, Hawaii, 1859 (abandoned) | |||
* ], destroyed in 1944 by the most recent eruption of ] during the Allies' occupation of southern ] (rebuilt) | |||
* Koae and ], were both destroyed by the same eruption of ] in January, 1960<ref>{{cite web |url=http://vhca.info/1959.htm |title=Article – Our Volcanic History by Gladys Flanders |publisher=Vhca.info |date=1959-11-15 |access-date=2013-12-08 |archive-date=2016-03-03 |archive-url=https://web.archive.org/web/20160303215410/http://vhca.info/1959.htm |url-status=dead }}</ref> (abandoned). | |||
* ], was destroyed by the eruption of the ] in 1990 (abandoned). | |||
* ], was largely inundated by lava in June 2018, with its subdivision ] being completely destroyed. | |||
===Towns damaged by lava flows=== | |||
* ], in the ]<ref name="EtnaAGU2004">{{cite book | title=Mount Etna:Volcano Laboratory | publisher=] (Geophysical Monograph 143) | editor=Bonaccorso, A. | year=2004 | location=Washington D.C. | page=3 | isbn=978-0-87590-408-5| display-editors=etal }}</ref> (rebuilt) | |||
* ], Samoa, by eruptions of ] between 1905 and 1911 | |||
* ], Italy, almost destroyed by the eruption of ] in 1928 (rebuilt)<ref name="Duncan_etal_1996">{{cite journal | title=The 1928 Eruption of Mount Etna Volcano, Sicily, and the Destruction of the Town of Mascali | first1=A.M. | last1=Duncan | first2=C. | last2=Dibben | first3=D.K. | last3=Chester | first4=J.E. |last4=Guest | journal=Disasters | year=1996 | volume=20 | issue=1 | pages=1–20 | doi=10.1111/j.1467-7717.1996.tb00511.x| pmid=8867507 | bibcode=1996Disas..20....1D }}</ref> | |||
* ] (village after which the volcano was named) and ], Mexico, by ] from 1943 to 1952 | |||
* ], in the 1973 ] eruption (rebuilt) | |||
* ], Reunion island, in 1977<ref name="Reunion1977">{{cite web | url=http://planet-terre.ens-lyon.fr/image-de-la-semaine/Img240-2008-06-23.xml | title=Église et gendarmerie envahies mais non détruites par la coulée d'avril 1977 de Piton Sainte Rose, île de La Réunion | publisher=] | work=Planet Terre | date=23 June 2008 | access-date=26 May 2018 | author=Thomas, Pierre | language=fr }}</ref> | |||
* ], by the eruption of ] in 1986–87 (abandoned) | |||
* ], ], in the eruption of ] in 2002<ref>{{cite web|url=https://volcano.si.edu/volcano.cfm?vn=223030|title=Global Volcanism Program - Nyiragongo|website=volcano.si.edu}}</ref> | |||
* Los Llanos de Aridane (] neighbourhood) and ] (El Paraíso neighbourhood) on ] in the ]<ref>{{Cite news|date=2021-09-25|title=La Palma volcano: Visual guide to what happened|language=en-GB|work=BBC News|url=https://www.bbc.com/news/world-europe-58681233|access-date=2021-09-25}}</ref><ref>{{Cite web|date=2021-09-23|title=Inge y Rainer, los dueños de la 'casa milagro' de La Palma: "Aunque no podemos ir, nos alivia que siga en pie"|url=https://www.elmundo.es/papel/historias/2021/09/23/614c645021efa01e388b4627.html|access-date=2021-09-25|quote=... en El Paraíso, justo la pedanía más afectada hasta la fecha por el río de lava del volcán. Más de la mitad de las casas, incluido el colegio local, ya han sido devoradas por la ceniza.|trans-quote=... in El Paraíso, just the district most affected to date by the river of lava from the volcano. More than half of the houses, including the local school, have already been consumed by ash.|website=El Mundo|language=es}}</ref><ref name="Todoque2021">{{cite news | url=https://www.elnacional.cat/es/sociedad/barrio-todoque-desaparece-totalmente-bajo-lava-volcan-la-palma_655610_102.html | title=El barrio de Todoque desaparece totalmente bajo la lava del volcán de La Palma | work=elnacional.cat | date=10 October 2021 | access-date=18 January 2022 | author=Sagrera, Berto | location=Barcelona | language=es}}</ref> | |||
===Towns destroyed by tephra=== | |||
] is lava in the form of ], ], ]s or ]s. | |||
* ], Italy, in the eruption of ] in 79 AD | |||
* ], Italy, in the eruption of ] in 79 AD | |||
* ], El Salvador, in the eruption of ] between 410 and 535 AD<ref>Bundschuh, J. and Alvarado, G. E (editors) (2007) ''Central America: Geology, Resources and Hazards'', volume 1, p. 56, London, Taylor and Francis</ref> | |||
* ], Indonesia, in the eruption of ] in 1815 | |||
* ], in 1995. Plymouth was the capital and only port of entry for ] and had to be completely abandoned, along with over half of the island. It is still the '']'' capital. | |||
== See also == | |||
{{Portal|Volcanoes|Geology}} | |||
* {{annotated link|Blue lava}} | |||
* {{annotated link|Lava planet}} | |||
* {{annotated link|Laze (geology)}} | |||
* {{annotated link|Vog}} | |||
==References== | |||
{{Reflist}} | |||
==External links== | ==External links== | ||
{{ |
{{Wiktionary|lava|aa|pahoehoe}} | ||
{{Commons and category|lava}} | |||
* | |||
* {{Cite EB1911 |wstitle=Lava |volume=16 |pages=289–290 |short=1}} | |||
* | |||
* | * | ||
* | |||
* | |||
* | |||
* | |||
* | |||
* {{Webarchive|url=https://web.archive.org/web/20160303171558/http://link.brightcove.com/services/link/bcpid687239786/bclid736245450/bctid736245427 |date=2016-03-03 }} Retrieved 23 August 2007 | |||
{{basalt}} | |||
] | |||
{{Authority control}} | |||
] | |||
] | |||
] | |||
] | |||
] | |||
] | |||
] | |||
] | |||
] | |||
] | ] | ||
] | |||
] | |||
] | |||
] | |||
] | |||
] | |||
] | |||
] | |||
] | |||
] | |||
] | |||
] | |||
] | |||
] | |||
] | |||
] | |||
] | |||
] | |||
] | |||
] | |||
] | |||
] |
Latest revision as of 03:59, 19 November 2024
Molten rock expelled by a volcano during an eruption "Lava flow" redirects here. For the programming anti-pattern, see Lava flow (programming). For other uses, see Lava (disambiguation).

Lava is molten or partially molten rock (magma) that has been expelled from the interior of a terrestrial planet (such as Earth) or a moon onto its surface. Lava may be erupted at a volcano or through a fracture in the crust, on land or underwater, usually at temperatures from 800 to 1,200 °C (1,470 to 2,190 °F). The volcanic rock resulting from subsequent cooling is also often called lava.
A lava flow is an outpouring of lava during an effusive eruption. (An explosive eruption, by contrast, produces a mixture of volcanic ash and other fragments called tephra, not lava flows.) The viscosity of most lava is about that of ketchup, roughly 10,000 to 100,000 times that of water. Even so, lava can flow great distances before cooling causes it to solidify, because lava exposed to air quickly develops a solid crust that insulates the remaining liquid lava, helping to keep it hot and inviscid enough to continue flowing.
Etymology
The word lava comes from Italian and is probably derived from the Latin word labes, which means a fall or slide. An early use of the word in connection with extrusion of magma from below the surface is found in a short account of the 1737 eruption of Vesuvius, written by Francesco Serao, who described "a flow of fiery lava" as an analogy to the flow of water and mud down the flanks of the volcano (a lahar) after heavy rain.
Properties of lava
Composition
Solidified lava on the Earth's crust is predominantly silicate minerals: mostly feldspars, feldspathoids, olivine, pyroxenes, amphiboles, micas and quartz. Rare nonsilicate lavas can be formed by local melting of nonsilicate mineral deposits or by separation of a magma into immiscible silicate and nonsilicate liquid phases.
Silicate lavas
Silicate lavas are molten mixtures dominated by oxygen and silicon, the most abundant elements of the Earth's crust, with smaller quantities of aluminium, calcium, magnesium, iron, sodium, and potassium and minor amounts of many other elements. Petrologists routinely express the composition of a silicate lava in terms of the weight or molar mass fraction of the oxides of the major elements (other than oxygen) present in the lava.
The silica component dominates the physical behavior of silicate magmas. Silicon ions in lava strongly bind to four oxygen ions in a tetrahedral arrangement. If an oxygen ion is bound to two silicon ions in the melt, it is described as a bridging oxygen, and lava with many clumps or chains of silicon ions connected by bridging oxygen ions is described as partially polymerized. Aluminium in combination with alkali metal oxides (sodium and potassium) also tends to polymerize the lava. Other cations, such as ferrous iron, calcium, and magnesium, bond much more weakly to oxygen and reduce the tendency to polymerize. Partial polymerization makes the lava viscous, so lava high in silica is much more viscous than lava low in silica.
Because of the role of silica in determining viscosity and because many other properties of a lava (such as its temperature) are observed to correlate with silica content, silicate lavas are divided into four chemical types based on silica content: felsic, intermediate, mafic, and ultramafic.
Felsic lava
Felsic or silicic lavas have a silica content greater than 63%. They include rhyolite and dacite lavas. With such a high silica content, these lavas are extremely viscous, ranging from 10 cP (10 Pa⋅s) for hot rhyolite lava at 1,200 °C (2,190 °F) to 10 cP (10 Pa⋅s) for cool rhyolite lava at 800 °C (1,470 °F). For comparison, water has a viscosity of about 1 cP (0.001 Pa⋅s). Because of this very high viscosity, felsic lavas usually erupt explosively to produce pyroclastic (fragmental) deposits. However, rhyolite lavas occasionally erupt effusively to form lava spines, lava domes or "coulees" (which are thick, short lava flows). The lavas typically fragment as they extrude, producing block lava flows. These often contain obsidian.
Felsic magmas can erupt at temperatures as low as 800 °C (1,470 °F). Unusually hot (>950 °C; >1,740 °F) rhyolite lavas, however, may flow for distances of many tens of kilometres, such as in the Snake River Plain of the northwestern United States.
Intermediate lava
Intermediate or andesitic lavas contain 52% to 63% silica, and are lower in aluminium and usually somewhat richer in magnesium and iron than felsic lavas. Intermediate lavas form andesite domes and block lavas and may occur on steep composite volcanoes, such as in the Andes. They are also commonly hotter than felsic lavas, in the range of 850 to 1,100 °C (1,560 to 2,010 °F). Because of their lower silica content and higher eruptive temperatures, they tend to be much less viscous, with a typical viscosity of 3.5 × 10 cP (3,500 Pa⋅s) at 1,200 °C (2,190 °F). This is slightly greater than the viscosity of smooth peanut butter. Intermediate lavas show a greater tendency to form phenocrysts. Higher iron and magnesium tends to manifest as a darker groundmass, including amphibole or pyroxene phenocrysts.
Mafic lava
Mafic or basaltic lavas are typified by relatively high magnesium oxide and iron oxide content (whose molecular formulas provide the consonants in mafic) and have a silica content limited to a range of 52% to 45%. They generally erupt at temperatures of 1,100 to 1,200 °C (2,010 to 2,190 °F) and at relatively low viscosities, around 10 to 10 cP (10 to 100 Pa⋅s). This is similar to the viscosity of ketchup, although it is still many orders of magnitude higher than that of water. Mafic lavas tend to produce low-profile shield volcanoes or flood basalts, because the less viscous lava can flow for long distances from the vent. The thickness of a solidified basaltic lava flow, particularly on a low slope, may be much greater than the thickness of the moving molten lava flow at any one time, because basaltic lavas may "inflate" by a continued supply of lava and its pressure on a solidified crust. Most basaltic lavas are of ʻaʻā or pāhoehoe types, rather than block lavas. Underwater, they can form pillow lavas, which are rather similar to entrail-type pahoehoe lavas on land.
Ultramafic lava
Ultramafic lavas, such as komatiite and highly magnesian magmas that form boninite, take the composition and temperatures of eruptions to the extreme. All have a silica content under 45%. Komatiites contain over 18% magnesium oxide and are thought to have erupted at temperatures of 1,600 °C (2,910 °F). At this temperature there is practically no polymerization of the mineral compounds, creating a highly mobile liquid. Viscosities of komatiite magmas are thought to have been as low as 100 to 1000 cP (0.1 to 1 Pa⋅s), similar to that of light motor oil. Most ultramafic lavas are no younger than the Proterozoic, with a few ultramafic magmas known from the Phanerozoic in Central America that are attributed to a hot mantle plume. No modern komatiite lavas are known, as the Earth's mantle has cooled too much to produce highly magnesian magmas.
Alkaline lavas
Some silicate lavas have an elevated content of alkali metal oxides (sodium and potassium), particularly in regions of continental rifting, areas overlying deeply subducted plates, or at intraplate hotspots. Their silica content can range from ultramafic (nephelinites, basanites and tephrites) to felsic (trachytes). They are more likely to be generated at greater depths in the mantle than subalkaline magmas. Olivine nephelinite lavas are both ultramafic and highly alkaline, and are thought to have come from much deeper in the mantle of the Earth than other lavas.
|
Tholeiitic basalt lava SiO2 (53.8%) Al2O3 (13.9%) FeO (9.3%) CaO (7.9%) MgO (4.1%) Na2O (3.0%) Fe2O3 (2.6%) TiO2 (2.0%) K2O (1.5%) P2O5 (0.4%) MnO (0.2%) |
Rhyolite lava SiO2 (73.2%) Al2O3 (14%) FeO (1.7%) CaO (1.3%) MgO (0.4%) Na2O (3.9%) Fe2O3 (0.6%) TiO2 (0.2%) K2O (4.1%) P2O5 (0.%) MnO (0.%) |
---|
Non-silicate lavas
Some lavas of unusual composition have erupted onto the surface of the Earth. These include:
- Carbonatite and natrocarbonatite lavas are known from Ol Doinyo Lengai volcano in Tanzania, which is the sole example of an active carbonatite volcano. Carbonatites in the geologic record are typically 75% carbonate minerals, with lesser amounts of silica-undersaturated silicate minerals (such as micas and olivine), apatite, magnetite, and pyrochlore. This may not reflect the original composition of the lava, which may have included sodium carbonate that was subsequently removed by hydrothermal activity, though laboratory experiments show that a calcite-rich magma is possible. Carbonatite lavas show stable isotope ratios indicating they are derived from the highly alkaline silicic lavas with which they are always associated, probably by separation of an immiscible phase. Natrocarbonatite lavas of Ol Doinyo Lengai are composed mostly of sodium carbonate, with about half as much calcium carbonate and half again as much potassium carbonate, and minor amounts of halides, fluorides, and sulphates. The lavas are extremely fluid, with viscosities only slightly greater than water, and are very cool, with measured temperatures of 491 to 544 °C (916 to 1,011 °F).
- Iron oxide lavas are thought to be the source of the iron ore at Kiruna, Sweden which formed during the Proterozoic. Iron oxide lavas of Pliocene age occur at the El Laco volcanic complex on the Chile-Argentina border. Iron oxide lavas are thought to be the result of immiscible separation of iron oxide magma from a parental magma of calc-alkaline or alkaline composition.
- Sulfur lava flows up to 250 metres (820 feet) long and 10 metres (33 feet) wide occur at Lastarria volcano, Chile. They were formed by the melting of sulfur deposits at temperatures as low as 113 °C (235 °F).
The term "lava" can also be used to refer to molten "ice mixtures" in eruptions on the icy satellites of the Solar System's giant planets.
Rheology

The lava's viscosity mostly determines the behavior of lava flows. While the temperature of common silicate lava ranges from about 800 °C (1,470 °F) for felsic lavas to 1,200 °C (2,190 °F) for mafic lavas, its viscosity ranges over seven orders of magnitude, from 10 cP (10 Pa⋅s) for felsic lavas to 10 cP (10 Pa⋅s) for mafic lavas. Lava viscosity is mostly determined by composition but also depends on temperature and shear rate.
Lava viscosity determines the kind of volcanic activity that takes place when the lava is erupted. The greater the viscosity, the greater the tendency for eruptions to be explosive rather than effusive. As a result, most lava flows on Earth, Mars, and Venus are composed of basalt lava. On Earth, 90% of lava flows are mafic or ultramafic, with intermediate lava making up 8% of flows and felsic lava making up just 2% of flows. Viscosity also determines the aspect (thickness relative to lateral extent) of flows, the speed with which flows move, and the surface character of the flows.
When highly viscous lavas erupt effusively rather than in their more common explosive form, they almost always erupt as high-aspect flows or domes. These flows take the form of block lava rather than ʻaʻā or pāhoehoe. Obsidian flows are common. Intermediate lavas tend to form steep stratovolcanoes, with alternating beds of lava from effusive eruptions and tephra from explosive eruptions. Mafic lavas form relatively thin flows that can move great distances, forming shield volcanoes with gentle slopes.
In addition to melted rock, most lavas contain solid crystals of various minerals, fragments of exotic rocks known as xenoliths, and fragments of previously solidified lava. The crystal content of most lavas gives them thixotropic and shear thinning properties. In other words, most lavas do not behave like Newtonian fluids, in which the rate of flow is proportional to the shear stress. Instead, a typical lava is a Bingham fluid, which shows considerable resistance to flow until a stress threshold, called the yield stress, is crossed. This results in plug flow of partially crystalline lava. A familiar example of plug flow is toothpaste squeezed out of a toothpaste tube. The toothpaste comes out as a semisolid plug, because shear is concentrated in a thin layer in the toothpaste next to the tube and only there does the toothpaste behave as a fluid. Thixotropic behavior also hinders crystals from settling out of the lava. Once the crystal content reaches about 60%, the lava ceases to behave like a fluid and begins to behave like a solid. Such a mixture of crystals with melted rock is sometimes described as crystal mush.
Lava flow speeds vary based primarily on viscosity and slope. In general, lava flows slowly, with typical speeds for Hawaiian basaltic flows of 0.40 km/h (0.25 mph) and maximum speeds of 10 to 48 km/h (6 to 30 mph) on steep slopes. An exceptional speed of 32 to 97 km/h (20 to 60 mph) was recorded following the collapse of a lava lake at Mount Nyiragongo. The scaling relationship for lavas is that the average speed of a flow scales as the square of its thickness divided by its viscosity. This implies that a rhyolite flow would have to be about a thousand times thicker than a basalt flow to flow at a similar speed.
Temperature

The temperature of most types of molten lava ranges from about 800 °C (1,470 °F) to 1,200 °C (2,190 °F) depending on the lava's chemical composition. This temperature range is similar to the hottest temperatures achievable with a forced air charcoal forge. Lava is most fluid when first erupted, becoming much more viscous as its temperature drops.
Lava flows quickly develop an insulating crust of solid rock as a result of radiative loss of heat. Thereafter, the lava cools by a very slow conduction of heat through the rocky crust. For instance, geologists of the United States Geological Survey regularly drilled into the Kilauea Iki lava lake, formed in an eruption in 1959. After three years, the solid surface crust, whose base was at a temperature of 1,065 °C (1,949 °F), was still only 14 m (46 ft) thick, even though the lake was about 100 m (330 ft) deep. Residual liquid was still present at depths of around 80 m (260 ft) nineteen years after the eruption.
A cooling lava flow shrinks, and this fractures the flow. Basalt flows show a characteristic pattern of fractures. The uppermost parts of the flow show irregular downward-splaying fractures, while the lower part of the flow shows a very regular pattern of fractures that break the flow into five- or six-sided columns. The irregular upper part of the solidified flow is called the entablature, while the lower part that shows columnar jointing is called the colonnade. (The terms are borrowed from Greek temple architecture.) Likewise, regular vertical patterns on the sides of columns, produced by cooling with periodic fracturing, are described as chisel marks. Despite their names, these are natural features produced by cooling, thermal contraction, and fracturing.
As lava cools, crystallizing inwards from its edges, it expels gases to form vesicles at the lower and upper boundaries. These are described as pipe-stem vesicles or pipe-stem amygdales. Liquids expelled from the cooling crystal mush rise upwards into the still-fluid center of the cooling flow and produce vertical vesicle cylinders. Where these merge towards the top of the flow, they form sheets of vesicular basalt and are sometimes capped with gas cavities that sometimes fill with secondary minerals. The beautiful amethyst geodes found in the flood basalts of South America formed in this manner.
Flood basalts typically crystallize little before they cease flowing, and, as a result, flow textures are uncommon in less silicic flows. On the other hand, flow banding is common in felsic flows.
Lava morphology

The morphology of lava describes its surface form or texture. More fluid basaltic lava flows tend to form flat sheet-like bodies, whereas viscous rhyolite lava flows form knobbly, blocky masses of rock. Lava erupted underwater has its own distinctive characteristics.

ʻAʻā
The following subsection is about the types of lava flow. For the sculpture of a god from the Pacific island of Rurutu, see Statue of A'a from Rurutu.
ʻAʻā (also spelled aa, aʻa, ʻaʻa, and a-aa, and pronounced [ʔəˈʔaː] or /ˈɑː(ʔ)ɑː/) is one of three basic types of flow lava. ʻAʻā is basaltic lava characterized by a rough or rubbly surface composed of broken lava blocks called clinker. The word is Hawaiian meaning "stony rough lava", but also to "burn" or "blaze"; it was introduced as a technical term in geology by Clarence Dutton.
The loose, broken, and sharp, spiny surface of an ʻaʻā flow makes hiking difficult and slow. The clinkery surface actually covers a massive dense core, which is the most active part of the flow. As pasty lava in the core travels downslope, the clinkers are carried along at the surface. At the leading edge of an ʻaʻā flow, however, these cooled fragments tumble down the steep front and are buried by the advancing flow. This produces a layer of lava fragments both at the bottom and top of an ʻaʻā flow.
Accretionary lava balls as large as 3 metres (10 feet) are common on ʻaʻā flows. ʻAʻā is usually of higher viscosity than pāhoehoe. Pāhoehoe can turn into ʻaʻā if it becomes turbulent from meeting impediments or steep slopes.
The sharp, angled texture makes ʻaʻā a strong radar reflector, and can easily be seen from an orbiting satellite (bright on Magellan pictures).
ʻAʻā lavas typically erupt at temperatures of 1,050 to 1,150 °C (1,920 to 2,100 °F) or greater.
Pāhoehoe

Pāhoehoe (also spelled pahoehoe, from Hawaiian [paːˈhoweˈhowe] meaning "smooth, unbroken lava") is basaltic lava that has a smooth, billowy, undulating, or ropy surface. These surface features are due to the movement of very fluid lava under a congealing surface crust. The Hawaiian word was introduced as a technical term in geology by Clarence Dutton.
A pāhoehoe flow typically advances as a series of small lobes and toes that continually break out from a cooled crust. It also forms lava tubes where the minimal heat loss maintains a low viscosity. The surface texture of pāhoehoe flows varies widely, displaying all kinds of bizarre shapes often referred to as lava sculpture. With increasing distance from the source, pāhoehoe flows may change into ʻaʻā flows in response to heat loss and consequent increase in viscosity. Experiments suggest that the transition takes place at a temperature between 1,200 and 1,170 °C (2,190 and 2,140 °F), with some dependence on shear rate. Pahoehoe lavas typically have a temperature of 1,100 to 1,200 °C (2,010 to 2,190 °F).
On the Earth, most lava flows are less than 10 km (6.2 mi) long, but some pāhoehoe flows are more than 50 km (31 mi) long. Some flood basalt flows in the geologic record extend for hundreds of kilometres.
The rounded texture makes pāhoehoe a poor radar reflector, and is difficult to see from an orbiting satellite (dark on Magellan picture).
Block lava flows

Block lava flows are typical of andesitic lavas from stratovolcanoes. They behave in a similar manner to ʻaʻā flows but their more viscous nature causes the surface to be covered in smooth-sided angular fragments (blocks) of solidified lava instead of clinkers. As with ʻaʻā flows, the molten interior of the flow, which is kept insulated by the solidified blocky surface, advances over the rubble that falls off the flow front. They also move much more slowly downhill and are thicker in depth than ʻaʻā flows.
Pillow lava
Main article: Pillow lava
Pillow lava is the lava structure typically formed when lava emerges from an underwater volcanic vent or subglacial volcano or a lava flow enters the ocean. The viscous lava gains a solid crust on contact with the water, and this crust cracks and oozes additional large blobs or "pillows" as more lava emerges from the advancing flow. Since water covers the majority of Earth's surface and most volcanoes are situated near or under bodies of water, pillow lava is very common.
Lava landforms
Because it is formed from viscous molten rock, lava flows and eruptions create distinctive formations, landforms and topographical features from the macroscopic to the microscopic.
Volcanoes
Main article: Volcano
Volcanoes are the primary landforms built by repeated eruptions of lava and ash over time. They range in shape from shield volcanoes with broad, shallow slopes formed from predominantly effusive eruptions of relatively fluid basaltic lava flows, to steeply-sided stratovolcanoes (also known as composite volcanoes) made of alternating layers of ash and more viscous lava flows typical of intermediate and felsic lavas.
A caldera, which is a large subsidence crater, can form in a stratovolcano, if the magma chamber is partially or wholly emptied by large explosive eruptions; the summit cone no longer supports itself and thus collapses in on itself afterwards. Such features may include volcanic crater lakes and lava domes after the event. However, calderas can also form by non-explosive means such as gradual magma subsidence. This is typical of many shield volcanoes.
Cinder and spatter cones
Main article: Volcanic coneCinder cones and spatter cones are small-scale features formed by lava accumulation around a small vent on a volcanic edifice. Cinder cones are formed from tephra or ash and tuff which is thrown from an explosive vent. Spatter cones are formed by accumulation of molten volcanic slag and cinders ejected in a more liquid form.
Kīpukas
Main article: KīpukaAnother Hawaiian English term derived from the Hawaiian language, a kīpuka denotes an elevated area such as a hill, ridge or old lava dome inside or downslope from an area of active volcanism. New lava flows will cover the surrounding land, isolating the kīpuka so that it appears as a (usually) forested island in a barren lava flow.
Lava domes and coulées
Main article: Lava dome
Lava domes are formed by the extrusion of viscous felsic magma. They can form prominent rounded protuberances, such as at Valles Caldera. As a volcano extrudes silicic lava, it can form an inflation dome or endogenous dome, gradually building up a large, pillow-like structure which cracks, fissures, and may release cooled chunks of rock and rubble. The top and side margins of an inflating lava dome tend to be covered in fragments of rock, breccia and ash.
Examples of lava dome eruptions include the Novarupta dome, and successive lava domes of Mount St Helens.
When a dome forms on an inclined surface it can flow in short thick flows called coulées (dome flows). These flows often travel only a few kilometres from the vent.
Lava tubes
Main article: Lava tubeLava tubes are formed when a flow of relatively fluid lava cools on the upper surface sufficiently to form a crust. Beneath this crust, which being made of rock is an excellent insulator, the lava can continue to flow as a liquid. When this flow occurs over a prolonged period of time the lava conduit can form a tunnel-like aperture or lava tube, which can conduct molten rock many kilometres from the vent without cooling appreciably. Often these lava tubes drain out once the supply of fresh lava has stopped, leaving a considerable length of open tunnel within the lava flow.
Lava tubes are known from the modern day eruptions of Kīlauea, and significant, extensive and open lava tubes of Tertiary age are known from North Queensland, Australia, some extending for 15 kilometres (9 miles).
Lava lakes
Main article: Lava lake
Rarely, a volcanic cone may fill with lava but not erupt. Lava which pools within the caldera is known as a lava lake. Lava lakes do not usually persist for long, either draining back into the magma chamber once pressure is relieved (usually by venting of gases through the caldera), or by draining via eruption of lava flows or pyroclastic explosion.
There are only a few sites in the world where permanent lakes of lava exist. These include:
- Mount Erebus, Antarctica
- Erta Ale, Ethiopia
- Nyiragongo, Democratic Republic of Congo
- Ambrym, Vanuatu.
Lava delta
Main article: Lava deltaLava deltas form wherever sub-aerial flows of lava enter standing bodies of water. The lava cools and breaks up as it encounters the water, with the resulting fragments filling in the seabed topography such that the sub-aerial flow can move further offshore. Lava deltas are generally associated with large-scale, effusive type basaltic volcanism.
Lava fountains
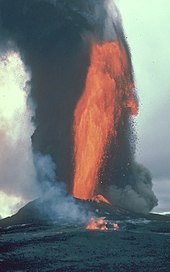
A lava fountain is a volcanic phenomenon in which lava is forcefully but non-explosively ejected from a crater, vent, or fissure. The highest lava fountain recorded was during the 23 November 2013 eruption of Mount Etna in Italy, which reached a stable height of around 2,500 m (8,200 ft) for 18 minutes, briefly peaking at a height of 3,400 m (11,000 ft). Lava fountains may occur as a series of short pulses, or a continuous jet of lava. They are commonly associated with Hawaiian eruptions.
Hazards
Lava flows are enormously destructive to property in their path. However, casualties are rare since flows are usually slow enough for people and animals to escape, though this is dependent on the viscosity of the lava. Nevertheless, injuries and deaths have occurred, either because they had their escape route cut off, because they got too close to the flow or, more rarely, if the lava flow front travels too quickly. This notably happened during the eruption of Nyiragongo in Zaire (now Democratic Republic of the Congo). On the night of 10 January 1977, a crater wall was breached and a fluid lava lake drained out in under an hour. The resulting flow sped down the steep slopes at up to 100 km/h (62 mph), and overwhelmed several villages while residents were asleep. As a result of this disaster, the mountain was designated a Decade Volcano in 1991.
Deaths attributed to volcanoes frequently have a different cause. For example, volcanic ejecta, pyroclastic flow from a collapsing lava dome, lahars, poisonous gases that travel ahead of lava, or explosions caused when the flow comes into contact with water. A particularly dangerous area is called a lava bench. This very young ground will typically break off and fall into the sea.
Areas of recent lava flows continue to represent a hazard long after the lava has cooled. Where young flows have created new lands, land is more unstable and can break off into the sea. Flows often crack deeply, forming dangerous chasms, and a fall against ʻaʻā lava is similar to falling against broken glass. Rugged hiking boots, long pants, and gloves are recommended when crossing lava flows.
Diverting a lava flow is extremely difficult, but it can be accomplished in some circumstances, as was once partially achieved in Vestmannaeyjar, Iceland. The optimal design of simple, low-cost barriers that divert lava flows is an area of ongoing research.
Towns destroyed by lava flows

- The Nisga'a villages of Lax Ksiluux and Wii Lax K'abit in northwestern British Columbia, Canada, were destroyed by thick lava flows during the eruption of Tseax Cone in the 1700s.
- Garachico on the island of Tenerife was destroyed by the eruption of Trevejo (1706) (rebuilt).
- Cagsawa, Philippines, buried by lava erupted from Mayon Volcano in 1814
- Keawaiki, Hawaii, 1859 (abandoned)
- San Sebastiano al Vesuvio, Italy, destroyed in 1944 by the most recent eruption of Mount Vesuvius during the Allies' occupation of southern Italy (rebuilt)
- Koae and Kapoho, Hawaii, were both destroyed by the same eruption of Kīlauea in January, 1960 (abandoned).
- Kalapana, Hawaii, was destroyed by the eruption of the Kīlauea volcano in 1990 (abandoned).
- Kapoho, Hawaii, was largely inundated by lava in June 2018, with its subdivision Vacationland Hawaii being completely destroyed.
Towns damaged by lava flows
- Catania, Italy, in the 1669 Etna eruption (rebuilt)
- Sale'aula, Samoa, by eruptions of Mt Matavanu between 1905 and 1911
- Mascali, Italy, almost destroyed by the eruption of Mount Etna in 1928 (rebuilt)
- Parícutin (village after which the volcano was named) and San Juan Parangaricutiro, Mexico, by Parícutin from 1943 to 1952
- Heimaey, Iceland, in the 1973 Eldfell eruption (rebuilt)
- Piton Sainte-Rose, Reunion island, in 1977
- Royal Gardens, Hawaii, by the eruption of Kilauea in 1986–87 (abandoned)
- Goma, Democratic Republic of Congo, in the eruption of Nyiragongo in 2002
- Los Llanos de Aridane (Todoque neighbourhood) and El Paso (El Paraíso neighbourhood) on La Palma in the 2021 Cumbre Vieja volcanic eruption
Towns destroyed by tephra
Tephra is lava in the form of volcanic ash, lapilli, volcanic bombs or volcanic blocks.
- Pompeii, Italy, in the eruption of Mount Vesuvius in 79 AD
- Herculaneum, Italy, in the eruption of Mount Vesuvius in 79 AD
- Cerén, El Salvador, in the eruption of Ilopango between 410 and 535 AD
- Sumbawa Island, Indonesia, in the eruption of Mount Tambora in 1815
- Plymouth, Montserrat, in 1995. Plymouth was the capital and only port of entry for Montserrat and had to be completely abandoned, along with over half of the island. It is still the de jure capital.
See also
- Blue lava – Optical phenomenon resulting from burning sulfur
- Lava planet – Terrestrial planet with the surface covered by molten lava
- Laze (geology) – Acid haze formed when molten lava enters the cold ocean
- Vog – Air pollution resulting from volcanic gases reacting with the atmosphere
References
- Philpotts, Anthony R.; Ague, Jay J. (2009). Principles of igneous and metamorphic petrology (2nd ed.). Cambridge, UK: Cambridge University Press. pp. 53–55. ISBN 9780521880060.
- "Lava". Merriam-Webster Online Dictionary. 2012-08-31. Retrieved 8 December 2013.
- "Lava". Dictionary.reference.com. 1994-12-07. Retrieved 8 December 2013.
- Serao, Francesco (1778). Istoria dell' incendio del Vesuvio accaduto nel mese di maggio dell'anno MDCCXXXVII. Naples: Presso Il De Bonis. Retrieved 6 August 2022.
- "Vesuvius Erupts, 1738". Linda Hall Library of Science, Engineering & Technology. Retrieved 6 August 2022.
- ^ Philpotts & Ague 2009, p. 19.
- ^ Guijón, R.; Henríquez, F.; Naranjo, J.A. (2011). "Geological, Geographical and Legal Considerations for the Conservation of Unique Iron Oxide and Sulphur Flows at El Laco and Lastarria Volcanic Complexes, Central Andes, Northern Chile". Geoheritage. 3 (4): 99–315. Bibcode:2011Geohe...3..299G. doi:10.1007/s12371-011-0045-x. S2CID 129179725.
- ^ Harlov, D.E.; et al. (2002). "Apatite–monazite relations in the Kiirunavaara magnetite–apatite ore, northern Sweden". Chemical Geology. 191 (1–3): 47–72. Bibcode:2002ChGeo.191...47H. doi:10.1016/s0009-2541(02)00148-1.
- Philpotts & Ague 2009, pp. 132–133.
- ^ Philpotts & Ague 2009, p. 25.
- Schmincke, Hans-Ulrich (2003). Volcanism. Berlin: Springer. p. 38. ISBN 9783540436508.
- Casq, R.A.F.; Wright, J.V. (1987). Volcanic Successions. Unwin Hyman Inc. p. 528. ISBN 978-0-04-552022-0.
- ^ Philpotts & Ague 2009, p. 23.
- Philpotts & Ague 2009, pp. 70–77.
- ^ Schmincke 2003, p. 132.
- ^ Philpotts & Ague 2009, p. 20.
- Bonnichsen, B.; Kauffman, D.F. (1987). "Physical features of rhyolite lava flows in the Snake River Plain volcanic province, southwestern Idaho". Geological Society of America Special Paper. Geological Society of America Special Papers. 212: 119–145. doi:10.1130/SPE212-p119. ISBN 0-8137-2212-8.
- Schmincke 2003, pp. 21–24, 132, 143.
- Philpotts & Ague 2009, pp. 23–611.
- Takeuchi, Shingo (5 October 2011). "Preeruptive magma viscosity: An important measure of magma eruptibility". Journal of Geophysical Research. 116 (B10): B10201. Bibcode:2011JGRB..11610201T. doi:10.1029/2011JB008243.
- Philpotts & Ague 2009, pp. 1376–377.
- Philpotts & Ague 2009, pp. 23–25.
- Philpotts & Ague 2009, p. 53-55, 59-64.
- ^ Schmincke 2003, pp. 128–132.
- Arndt, N.T. (1994). "Archean komatiites". In Condie, K.C. (ed.). Archean Crustal Evolution. Amsterdam: Elsevier. p. 19. ISBN 978-0-444-81621-4.
- Philpotts & Ague 2009, pp. 399–400.
- Philpotts & Ague 2009, pp. 139–148.
- Philpotts & Ague 2009, pp. 606–607.
- "Stikine Volcanic Belt: Volcano Mountain". Catalogue of Canadian volcanoes. Archived from the original on 2009-03-07. Retrieved 23 November 2007.
- Philpotts & Ague 2009, p. 145.
- Vic Camp, How volcanoes work, Unusual Lava Types Archived 2017-10-23 at the Wayback Machine, San Diego State University, Geology
- Philpotts & Ague 2009, pp. 396–397.
- Keller, Jörg; Krafft, Maurice (November 1990). "Effusive natrocarbonatite activity of Oldoinyo Lengai, June 1988". Bulletin of Volcanology. 52 (8): 629–645. Bibcode:1990BVol...52..629K. doi:10.1007/BF00301213. S2CID 129106033.
- McBride; Gilmore, eds. (2007). An introduction to the Solar System. Cambridge University Press. p. 392.
- ^ Sonder, I; Zimanowski, B; Büttner, R (2006). "Non-Newtonian viscosity of basaltic magma". Geophysical Research Letters. 330 (2): L02303. Bibcode:2006GeoRL..33.2303S. doi:10.1029/2005GL024240.
- Schmincke 2003, p. 128.
- ^ "Lava Flows" (PDF). UMass Department of Geosciences. University of Massachusetts Amherst. 11 February 2004. p. 19. Retrieved 5 June 2018.
- Peterson, Donald W.; Tilling, Robert I. (May 1980). "Transition of basaltic lava from pahoehoe to aa, Kilauea Volcano, Hawaii: Field observations and key factors". Journal of Volcanology and Geothermal Research. 7 (3–4): 271–293. Bibcode:1980JVGR....7..271P. doi:10.1016/0377-0273(80)90033-5.
- ^ Schmincke 2003, pp. 132–138.
- Schmincke 2003, pp. 143–144.
- Schmincke 2003, pp. 127–128.
- Pinkerton, H.; Bagdassarov, N. (2004). "Transient phenomena in vesicular lava flows based on laboratory experiments with analogue materials". Journal of Volcanology and Geothermal Research. 132 (2–3): 115–136. Bibcode:2004JVGR..132..115B. doi:10.1016/s0377-0273(03)00341-x.
- Schmincke 2003, pp. 39–40.
- Philpotts & Ague 2009, p. 40.
- Philpotts & Ague 2009, p. 16.
- Philpotts & Ague 2009, p. 71.
- Cheng, Zhilong; Yang, Jian; Zhou, Lang; Liu, Yan; Wang, Qiuwang (January 2016). "Characteristics of charcoal combustion and its effects on iron-ore sintering performance". Applied Energy. 161: 364–374. Bibcode:2016ApEn..161..364C. doi:10.1016/j.apenergy.2015.09.095.
- Philpotts & Ague 2009, pp. 55–56.
- Philpotts & Ague 2009, pp. 58–59.
- Philpotts & Ague 2009, p. 48.
- Philpotts & Ague 2009, p. 72.
- "ʻaʻā". Hawaiian Dictionary (Hwn to Eng). Archived from the original on 28 December 2012.
- ^ Kemp, James Furman (1918). A handbook of rocks for use without the microscope : with a glossary of the names of rocks and other lithological terms. Vol. 5. New York: D. Van Nostrand. pp. 180, 240.
- ^ Dutton, C. E. (1883). "Hawaiian volcanoes". Annual Report U.S. Geological Survey. 4 (95): 240.
- ^ Schmincke 2003, pp. 131–132.
- Macdonald, Gordon A.; Abbott, Agatin T.; Peterson, Frank L. (1983). Volcanoes in the sea : the geology of Hawaii (2nd ed.). Honolulu: University of Hawaii Press. p. 23. ISBN 0824808320.
- ^ McGounis-Mark, Peter. "Radar Studies of Lava Flows". Volcanic Features of Hawaii and Other Worlds. Lunar and Planetary Institute. Retrieved 18 March 2017.
- Pinkerton, Harry; James, Mike; Jones, Alun (March 2002). "Surface temperature measurements of active lava flows on Kilauea volcano, Hawai'i". Journal of Volcanology and Geothermal Research. 113 (1–2): 159–176. Bibcode:2002JVGR..113..159P. doi:10.1016/S0377-0273(01)00257-8.
- Cigolini, Corrado; Borgia, Andrea; Casertano, Lorenzo (March 1984). "Intra-crater activity, aa-block lava, viscosity and flow dynamics: Arenal Volcano, Costa Rica". Journal of Volcanology and Geothermal Research. 20 (1–2): 155–176. Bibcode:1984JVGR...20..155C. doi:10.1016/0377-0273(84)90072-6.
- "pāhoehoe". Hawaiian Dictionary (Hwn to Eng). Archived from the original on 18 September 2012.
- Sehlke, A.; Whittington, A.; Robert, B.; Harris, A.; Gurioli, L.; Médard, E. (17 October 2014). "Pahoehoe to 'a'a transition of Hawaiian lavas: an experimental study". Bulletin of Volcanology. 76 (11): 876. doi:10.1007/s00445-014-0876-9. S2CID 129019507.
- "Types and Processes Gallery: Lava Flows". Global Volcanism Program. Smithsonian Institution. 2013. Retrieved 1 December 2015.
- Philpotts & Ague 2009, p. 53.
- Lewis, J.V. (1914). "Origin of pillow lavas". Bulletin of the Geological Society of America. 25 (1): 639. Bibcode:1914GSAB...25..591L. doi:10.1130/GSAB-25-591.
- Philpotts & Ague 2009, pp. 59–73.
- Schmincke 2003, pp. 147–148.
- Schmincke 2003, pp. 132, 286.
- Schmincke 2003, pp. 149–151.
- Macdonald, Abbott & Peterson 1983, pp. 26–17.
- Macdonald, Abbott & Peterson 1983, pp. 22–23.
- Schmincke 2003, pp. 132–138, 152–153.
- Schmincke 2003, pp. 132–134.
- Macdonald, Abbott & Peterson 1983, pp. 23, 26–29.
- Macdonald, Abbott & Peterson 1983, p. 27.
- Atkinson, A.; Griffin, T. J.; Stephenson, P. J. (June 1975). "A major lava tube system from Undara Volcano, North Queensland". Bulletin Volcanologique. 39 (2): 266–293. Bibcode:1975BVol...39..266A. doi:10.1007/BF02597832. S2CID 129126355.
- Schmincke 2003, p. 27.
- ^ Lev, Einat; Ruprecht, Philipp; Oppenheimer, Clive; Peters, Nial; Patrick, Matt; Hernández, Pedro A.; Spampinato, Letizia; Marlow, Jeff (September 2019). "A global synthesis of lava lake dynamics". Journal of Volcanology and Geothermal Research. 381: 16–31. Bibcode:2019JVGR..381...16L. doi:10.1016/j.jvolgeores.2019.04.010. S2CID 182844266.
- Philpotts & Ague 2009, p. 61.
- Burgi, P.-Y.; Darrah, T. H.; Tedesco, D.; Eymold, W. K. (May 2014). "Dynamics of the Mount Nyiragongo lava lake: DYNAMICS OF THE MT. NYIRAGONGO LAVA LAKE". Journal of Geophysical Research: Solid Earth. 119 (5): 4106–4122. doi:10.1002/2013JB010895.
- Bosman, Alessandro; Casalbore, Daniele; Romagnoli, Claudia; Chiocci, Francesco Latino (July 2014). "Formation of an 'a'ā lava delta: insights from time-lapse multibeam bathymetry and direct observations during the Stromboli 2007 eruption". Bulletin of Volcanology. 76 (7): 838. Bibcode:2014BVol...76..838B. doi:10.1007/s00445-014-0838-2. S2CID 129797425.
- Bonaccorso, A.; Calvari, S.; Linde, A.; Sacks, S. (28 July 2014). "Eruptive processes leading to the most explosive lava fountain at Etna volcano: The 23 November 2013 episode". Geophysical Research Letters. 41 (14): 4912–4919. Bibcode:2014GeoRL..41.4912B. doi:10.1002/2014GL060623. S2CID 129813334.
To the best of our knowledge, it reached the highest value ever measured for a lava fountain on Earth.
- Macdonald, Abbott & Peterson 1983, p. 9.
- ^ Lava Flows and Their Effects USGS
- Nyiragongo – Could it happen here? USGS Hawaiian Volcano Observatory
- Sonstroem, Eric (14 September 2010). "Vestmannaeyjar, The Town That Fought A Volcano And Won". indianapublicmedia.org. Indiana Public Media. Archived from the original on 23 February 2017. Retrieved 24 November 2017.
- Dietterich, Hannah; Cashman, Katherine; Rust, Alison; Lev, Einat (2015). "Diverting lava flows in the lab". Nature Geoscience. 8 (7): 494–496. Bibcode:2015NatGe...8..494D. doi:10.1038/ngeo2470.
- Hinton, Edward; Hogg, Andrew; Huppert, Herbert (2020). "Viscous free-surface flows past cylinders". Physical Review Fluids. 5 (84101): 084101. Bibcode:2020PhRvF...5h4101H. doi:10.1103/PhysRevFluids.5.084101. hdl:1983/f52f7078-5936-4e37-9d79-be456f08eb5c. S2CID 225416948.
- "Tourist attractions of Albay Province, Philippines". Nscb.gov.ph. Archived from the original on 2016-09-21. Retrieved 2013-12-08.
- "Article – Our Volcanic History by Gladys Flanders". Vhca.info. 1959-11-15. Archived from the original on 2016-03-03. Retrieved 2013-12-08.
- Bonaccorso, A.; et al., eds. (2004). Mount Etna:Volcano Laboratory. Washington D.C.: American Geophysical Union (Geophysical Monograph 143). p. 3. ISBN 978-0-87590-408-5.
- Duncan, A.M.; Dibben, C.; Chester, D.K.; Guest, J.E. (1996). "The 1928 Eruption of Mount Etna Volcano, Sicily, and the Destruction of the Town of Mascali". Disasters. 20 (1): 1–20. Bibcode:1996Disas..20....1D. doi:10.1111/j.1467-7717.1996.tb00511.x. PMID 8867507.
- Thomas, Pierre (23 June 2008). "Église et gendarmerie envahies mais non détruites par la coulée d'avril 1977 de Piton Sainte Rose, île de La Réunion". Planet Terre (in French). ENS de Lyon. Retrieved 26 May 2018.
- "Global Volcanism Program - Nyiragongo". volcano.si.edu.
- "La Palma volcano: Visual guide to what happened". BBC News. 2021-09-25. Retrieved 2021-09-25.
- "Inge y Rainer, los dueños de la 'casa milagro' de La Palma: "Aunque no podemos ir, nos alivia que siga en pie"". El Mundo (in Spanish). 2021-09-23. Retrieved 2021-09-25.
... en El Paraíso, justo la pedanía más afectada hasta la fecha por el río de lava del volcán. Más de la mitad de las casas, incluido el colegio local, ya han sido devoradas por la ceniza.
[... in El Paraíso, just the district most affected to date by the river of lava from the volcano. More than half of the houses, including the local school, have already been consumed by ash.] - Sagrera, Berto (10 October 2021). "El barrio de Todoque desaparece totalmente bajo la lava del volcán de La Palma". elnacional.cat (in Spanish). Barcelona. Retrieved 18 January 2022.
- Bundschuh, J. and Alvarado, G. E (editors) (2007) Central America: Geology, Resources and Hazards, volume 1, p. 56, London, Taylor and Francis
External links
- "Lava" . Encyclopædia Britannica. Vol. 16 (11th ed.). 1911. pp. 289–290.
- USGS definition of ʻAʻā
- USGS definition of Pāhoehoe
- USGS definition of Ropy Pāhoehoe
- Volcanic landforms of Hawaii
- USGS hazards associated with lava flows
- Hawaiian Volcano Observatory Volcano Watch newsletter article on Nyiragongo eruptions, 31 January 2002
- National Geographic lava video Archived 2016-03-03 at the Wayback Machine Retrieved 23 August 2007
Types of basalts | |
---|---|
Basalts by tectonic setting | |
Basalts by form and flow | |
Basalts by chemistry | |
Important minerals | |
![]() |