Revision as of 22:32, 29 November 2007 editRussBot (talk | contribs)Bots1,405,761 editsm Robot-assisted fix links to disambiguation page Ray← Previous edit | Revision as of 15:01, 3 December 2007 edit undoMax rspct (talk | contribs)Extended confirmed users11,122 edits →Multiple impact event: accuracy - not general acceptionNext edit → | ||
Line 159: | Line 159: | ||
Several other craters also appear to have been formed about the time of the K–T boundary. This suggests the possibility of near simultaneous multiple impacts, perhaps from a fragmented asteroidal object, similar to the ] cometary impact with ]. Among these are the ], a {{km to mi|24}} diameter impact crater in the ] {{nowrap|(65.17 ± 0.64 mya);}} the ], a {{km to mi|20}} diameter impact crater in the ] {{nowrap|(60–65 mya).}} Any other craters that might have formed in the ] would have been obscured by tectonic events like the relentless northward drift of Africa and India.<ref>{{cite journal|author=Mullen, L|date= ] ]|title= Debating the Dinosaur Extinction| url= http://www.astrobio.net/news/article1243.html|journal=Astrobiology Magazine|accessdate=2007-07-11}}</ref><ref>{{cite journal|author=Mullen, L| date= ], ] | title=Multiple impacts|url=http://www.astrobio.net/news/article1253.html|journal=Astrobiology Magazine|accessdate=2007-07-11}}</ref><ref name="Mullen Shiva">{{cite journal|author=Mullen, L|date=] ]|title=Shiva: Another K–T impact?|url=http://www.astrobio.net/news/article1281.html|journal=Astrobiology Magazine|accessdate=2007-07-11}}</ref> | Several other craters also appear to have been formed about the time of the K–T boundary. This suggests the possibility of near simultaneous multiple impacts, perhaps from a fragmented asteroidal object, similar to the ] cometary impact with ]. Among these are the ], a {{km to mi|24}} diameter impact crater in the ] {{nowrap|(65.17 ± 0.64 mya);}} the ], a {{km to mi|20}} diameter impact crater in the ] {{nowrap|(60–65 mya).}} Any other craters that might have formed in the ] would have been obscured by tectonic events like the relentless northward drift of Africa and India.<ref>{{cite journal|author=Mullen, L|date= ] ]|title= Debating the Dinosaur Extinction| url= http://www.astrobio.net/news/article1243.html|journal=Astrobiology Magazine|accessdate=2007-07-11}}</ref><ref>{{cite journal|author=Mullen, L| date= ], ] | title=Multiple impacts|url=http://www.astrobio.net/news/article1253.html|journal=Astrobiology Magazine|accessdate=2007-07-11}}</ref><ref name="Mullen Shiva">{{cite journal|author=Mullen, L|date=] ]|title=Shiva: Another K–T impact?|url=http://www.astrobio.net/news/article1281.html|journal=Astrobiology Magazine|accessdate=2007-07-11}}</ref> | ||
In September 2007, a team made up of scientists from the ] and the ] found evidence of an asteroid breakup of the parent-body of ], which is conjectured to have occurred about 160 mya. They believe this event caused the Chicxulub crater on Earth, and the ] on the Moon. | In September 2007, a team made up of scientists from the ] and the ] found evidence of an asteroid breakup of the parent-body of ], which is conjectured to have occurred about 160 mya. They believe this event may have caused the Chicxulub crater on Earth, and the ] on the Moon. | ||
===Maastrichtian sea-level regression=== | ===Maastrichtian sea-level regression=== |
Revision as of 15:01, 3 December 2007



The Cretaceous–Tertiary extinction event was the large-scale mass extinction of animal and plant species in a geologically short period of time, approximately 65.5 million years ago (mya). It is widely known as the K–T extinction event and is associated with a geological signature, usually a thin band dated to that time and found in various parts of the world, known as the K–T boundary. K is the traditional abbreviation for the Cretaceous Period, and T is the abbreviation for the Tertiary Period. The event marks the end of the Mesozoic Era, and the beginning of the Cenozoic Era.
Non-avian dinosaur fossils are only found below the K–T boundary and became extinct immediately before or during the event. A very small number of dinosaur fossils have been found above the K-T boundary, but they have been explained as reworked, that is, fossils that have been eroded from their original locations then preserved in later sedimentary layers. Mosasaurs, plesiosaurs, pterosaurs and many species of plants and invertebrates also became extinct. Mammalian and bird clades passed through the boundary with few extinctions, and radiation from those Maastrichtian clades occurred well past the boundary. Rates of extinction and radiation varied across different clades of organisms.
Many scientists theorize that the K-T extinctions were caused by one or more catastrophic geological events such as massive asteroid impacts or increased volcanic activity. Several impact craters and massive volcanic activity in the Deccan traps have been dated to the approximate time of the extinction event. These geological events may have reduced sunlight and hindered photosynthesis, leading to a massive disruption in Earth's ecology. Other researchers believe the extinction was more gradual, resulting from slower changes in sea level or climate.
Extinction patterns
There was a progressive decline in biodiversity during the Maastrichtian stage of the Cretaceous Period prior to the suggested ecological crisis induced by events at the K-T boundary. Furthermore, biodiversity required a substantial amount of time to recover from the K-T event, despite the probable existence of an abundance of vacant ecological niches.
Despite the severity of this boundary event, there was significant variability in the rate of extinction between and within different clades. Species which depended on photosynthesis declined or became extinct because of the reduction in solar energy reaching the earth's surface due to atmospheric particles blocking the sunlight. As is the case today, photosynthesizing organisms, such as phytoplankton and land plants, formed the primary part of the food chain in the late Cretaceous. Evidence suggests that herbivorous animals, which depended on plants and plankton as their food, died out as their food sources became scarce; consequently, top predators such as Tyrannosaurus rex also perished.
Coccolithophorids and molluscs, including ammonites, rudists, freshwater snails and mussels, as well as organisms whose food chain included these shell builders, became extinct or suffered heavy losses. For example, it is thought that ammonites were the principal food of mosasaurs, a group of giant marine reptiles that became extinct at the boundary.
Omnivores, insectivores and carrion-eaters survived the extinction event, perhaps because of the increased availability of their food sources. At the end of the Cretaceous there seem to have been no purely herbivorous or carnivorous mammals. Mammals and birds which survived the extinction fed on insects, larvae, worms, and snails, which in turn fed on dead plant and animal matter. Scientists theorise that these organisms survived the collapse of plant-based food chains because they fed on detritus.
In stream communities, few groups of animals became extinct. Stream communities rely less on food from living plants and more on detritus that washes in from land. This particular ecological niche buffered them from extinction. Similar, but more complex patterns have been found in the oceans. Extinction was more severe among animals living in the water column, than among animals living on or in the sea floor. Animals in the water column are almost entirely dependent on primary production from living phytoplankton, while animals living on or in the ocean floor feed on detritus or can switch to detritus feeding.
The largest air-breathing survivors of the event, crocodilians and champsosaurs, were semi-aquatic and had access to detritus. Modern crocodilians can live as scavengers and can survive for months without food, and their young are small, grow slowly, and feed largely on invertebrates and dead organisms or fragments of organisms for their first few years. These characteristics have been linked to crocodilian survival at the end of the Cretaceous.
Microbiota
The K–T boundary represents one of the most dramatic turnovers in the fossil record for various calcareous nanoplankton (which formed the calcium deposits that gave the Cretaceous its name). The turnover in this group is clearly marked at the species level. Statistical analysis of marine losses at this time suggests that the decrease in diversity was caused more by a sharp increase in extinctions than by a decrease in speciation. The K–T boundary record of dinoflagellates is not as well-understood, mainly because only cysts provide a fossil record, and not all dinoflagellate species have cyst-forming stages, thereby likely causing diversity to be underestimated. Recent studies indicate that there were no major shifts in dinoflagellates through the boundary layer.
Radiolaria have left a geological record since at least the Ordovician times, and their mineral fossil skeletons can be tracked across the K-T boundary. There is no evidence of mass extinction of these organisms, and, there is support for high productivity of these species in Southern high latitudes as a result of cooling temperatures in the early Paleocene. Approximately 46% of diatom species survived the transition from the Cretaceous to the Upper Paleocene. This suggests a significant turnover in species, but not a catastrophic extinction of diatoms, across the K–T boundary.
The occurrence of Planktonic foraminifera across the K-T boundary has been studied since the 1930s. Research spurred by the possibility of an impact event at the K-T boundary resulted in numerous publications detailing planktonic foraminiferal extinction at the boundary. However, there is debate ongoing between groups that believe the evidence indicates substantial extinction of these species at the K-T boundary, and those who believe the evidence supports multiple extinctions and expansions through the boundary.
As the biomass in the ocean is thought to have decreased during the hypothesised K-T event, numerous species of benthic foraminifera went extinct, presumably since they depend on organic debris for nutrients. However, as the marine microbiota recovered, it is thought that increased speciation of benthic foraminifera resulted from the increase in food sources. Phytoplankton recovery in the early Paleocene provided the food source to support large benthic foraminiferal assemblages, which are mainly detritus-feeding. Ultimate recovery of the benthic populations occurred over several stages lasting several hundred thousand years into the early Paleocene.
Marine invertebrates

There is variability in the fossil record as to the extinction rate of marine invertebrates across the K-T boundary. The apparent rate is influenced by the lack of fossil records rather than actual extinction.
Ostracodes, a class of small crustaceans that were prevalent in the upper Maastrichtian, left fossil deposits in a variety of locations. A review of these fossils shows that ostracode diversity was lower in the Paleocene than any other time in the Tertiary. However, current research cannot ascertain whether the extinctions occurred prior to or during the boundary interval itself.
Approximately 60% of late-Cretaceous Scleractinia coral genera failed to cross the K-T boundary into the Paleocene. Further analysis of the coral extinctions show that approximately 98% of colonial species, ones that inhabit warm, shallow tropical waters, went extinct. The solitary corals, which generally do not form reefs and inhabit colder and areas of the ocean below the photic zone were less impacted by the K-T boundary. Colonial coral species rely upon symbiosis with photosynthetic algae, which collapsed due to the events surrounding the K-T boundary. However, the use of data from coral fossils to support K-T extinction and subsequent Paleocene recovery must be weighed against the changes that occurred in coral ecosystems through the K-T boundary.
Most species of brachiopods, a small phylum of nearly extinct marine invertebrates, survived the K-T event and diversified during the early Paleocene. The numbers of cephalopod, echinoderm, bivalve, and brachiopod genera exhibited significant diminution after the K-T boundary.
Except for nautiloids (represented by the modern order Nautilida) and coleoids (which had already diverged into modern octopi, squids, and cuttlefish) all other species of the molluscan class Cephalopoda went extinct at the K-T boundary. These included the ecologically significant belemnoids, as well as the ammonoids, a group of highly diverse, numerous, and widely distributed shelled cephalopods. Researchers have pointed out that the reproductive strategy of the surviving nautiloids, which rely upon few and larger eggs, played a role in outsurviving their ammonoid counterparts through the extinction event. The ammonoids utilized a planktonic strategy of reproduction (numerous eggs and planktonic larvae), which would have been devastated by the K-T boundary event. Additional research has shown that subsequent to this elimination of ammonoids from the global biota, nautiloids began an evolutionary radiation into shell shapes and complexities theretofore known only from ammonoids.
Approximately 35% of echinoderm genera went extinct at the K-T boundary, although taxa that thrived in low-latitude, shallow-water environments during late Cretaceous had the highest extinction rate. Mid-latitude, deep-water echinoderms were much less affected at the K-T boundary. The pattern of extinction points to habitat loss, specifically the drowning of carbonate platforms, the shallow-water reefs in existence at that time, by the extinction event.
Other invertebrate groups, including rudists (reef-building clams) and inoceramids (giant relatives of modern scallops), also became completely extinct at the K-T boundary.
Fish

There are substantial fossil records of jawed fishes across the K–T boundary, which provides good evidence of extinction patterns of these classes of marine vertebrates. Within cartilaginous fish, approximately 80% of the sharks, rays, and skates families survived the extinction event, and fewer than 10% of teleost fish (bony fish) families became extinct. There is evidence of a mass kill of bony fishes at a fossil site immediately above the K-T boundary layer on Seymour Island near the Antarctica. It is speculated that fish were undergoing environmental stresses and K-T boundary event may have precipitated the mass extinction. However, the marine and freshwater environments of fishes mitigated environmental effects of the extinction event.
Terrestrial invertebrates
Insect damage to the fossilized leaves of flowering plants from fourteen sites in North America were used as a proxy for insect diversity across the K–T boundary and analyzed to determine the rate of extinction. Researchers found that Cretaceous sites, prior to the extinction event, had rich plant and insect-feeding diversity. However, during the early Paleocene, flora were relatively diverse with little predation from insects, even 1.7 million years after the extinction event.
Terrestrial plants
There is overwhelming evidence of global disruption of vegetation at the K-T boundary. However, there are important regional differences in the signature of vegetation turnover. In North America, the data suggest massive devastation and mass extinction of plants at the K-T boundary sections, although there were substantial megafloral changes before the boundary.
In high southern hemisphere latitudes, such as New Zealand and Antarctica mass-kill of vegetation caused no significant turnover in species, but dramatic and short-term changes in the relative abundance of plant groups. In North America, approximately 57% of plant species became extinct. The Paleocene recovery of plants began with a fern spike which signals the recovery from natural disasters, as was observed after the 1980 Mount St. Helens eruption.
Due to the wholesale destruction of vegetation at the K–T boundary, there was a significant proliferation of saprophytic organisms such as fungi which do not require photosynthesis and need organic substrates provided by the dead vegetation. The dominance of fungal species would have lasted only a few years until ferns and other plant species recovered when the atmosphere cleared and once again favored photosynthetic organisms.
Amphibians
There is no evidence of K–T boundary mass extinctions of amphibians, and there is strong evidence that most amphibians survived the event relatively unscathed. Several in-depth studies of salamander genera in fossil beds in Montana show that six of seven genera were unchanged after the event.
Frog species appear to have survived into the Paleocene with few species becoming extinct. However, the fossil record for frog families and genera is uneven. An extensive survey of three genera of frogs in Montana show that they were unaffected by the K–T event and survived apparently unchanged. The data show little or no evidence for extinction of amphibian families that bracket the K–T event. Amphibian survival resulted from the clade's ability to seek shelter in water or to build burrows in sediments, soil, wood, or beneath rocks.
Non-archosaur reptiles
The two living non-archosaurian reptile taxa, testudines (turtles) and lepidosaurs (snakes, lizards, and worm lizards), along with champsosaurs (semi-aquatic archosauromorphs which died out in the early Miocene), survived through the K–T boundary. Over 80% of Cretaceous turtle species passed through the K-T boundary. Additionally, all six turtle families in existence at the end of the Cretaceous survived into the Tertiary and are represented by current species.
Living lepidosaurs include Rhynchocephalia and Squamata. The Rhynchocephalia, or tuatara, were widespread and relatively successful group of lepidosaurs in the early Mesozoic, but began to decline by the mid-Cretaceous. They are represented today by a single genus located exclusively in New Zealand.
The order, Squamata, which is represented today by lizards, snakes, and amphisbaenia, radiated into various ecological niches during the Jurassic and were successful throughout the Cretaceous. They survived through the K-T boundary and are currently the most successful and diverse group of living reptiles with more than 6000 extant species. All living families of lizards represent survivors from the Cretaceous, so it is presumed that few went extinct at the boundary. No known family of terrestrial squamates went extinct at the boundary, and based on fossil evidence, they did not suffer any significant decline in numbers. Their small size, adaptable metabolism and ability to move to more favorable habitats were key factors to their survivability during the late Cretaceous and early Paleocene.
Marine reptiles including mosasaurs and plesiosaurs, giant aquatic reptiles which were the top marine predators, were extinct by the end of the Cretaceous.
Archosaurs
The archosaur clade includes two living orders, crocodilians (of which Alligatoridae, Crocodylidae and Gavialidae are the only surviving families) and birds, along with the extinct dinosaurs and pterosaurs.
Crocodilians
Ten crocodile families are represented in the Maastrichtian fossil records, of which five died out prior to the K-T boundary. Five families have both Maastrichtian and Paleocene fossil representatives. All of the surviving families of crocodilians inhabited freshwater and terrestrial environments, except for the Dyrosauridae which lived in freshwater and marine locations. Approximately 50% of crocodilian representatives survived across the K-T boundary, the only apparent trend being that no large crocodiles, such as the giant North American crocodile Deinosuchus, survived. Crocodilian survivability across the boundary may have resulted from their aquatic niche and ability to burrow, which reduced susceptibility to negative environmental effects at the boundary.
Pterosaurs
Only one family of pterosaurs, Azhdarchidae, is definitely present in the Maastrichtian, and it went extinct at the K-T boundary. These large pterosaurs were the last representatives of a group that contained 10 families during the mid-Cretaceous. Smaller pterosaurs went extinct prior to the Maastrichtian as a result of competition with birds that filled the same ecological niche and as a result of their non-sheltering environment niche.
Birds
Most paleontologists regard birds as the only surviving dinosaurs (see Origin of birds). However, all non-neornithean birds became extinct, including flourishing groups like enantiornithines and hesperornithiforms. Several analyses of bird fossils show divergence of species prior to the K-T boundary, and that duck, chicken and ratite bird relatives existed with non-avian dinosaurs. Neornithine birds survived the K-T boundary as a result of their abilities to dive, swim, or seek shelter in water, marshlands. Many species of birds can build burrows, nest in tree holes, or termite nests, all of which provided shelter from the environmental effects at the K-T boundary. Long-term survival past the boundary was assured as a result of filling ecological niches left empty by extinction of dinosaurs.
Dinosaurs
More has been published about the extinction of dinosaurs at the K-T boundary than any other group of organisms. Except for a few controversial claims, all non-avian dinosaurs went extinct at the K-T boundary. The dinosaur fossil record has been interpreted to show both a decline in diversity and no decline in diversity during the last few million years of the Cretaceous, and it may be that the quality of the dinosaur fossil record is simply not good enough to permit researchers to distinguish between the choices. Since there is no evidence that late Maastrichtian nonavian dinosaurs could burrow, swim or dive, they were unable to shelter themselves from the worst parts of any environmental stress that occurred at the K-T boundary. It is possible that small dinosaurs (other than birds) did survive, but they would have been deprived of food as both herbivorous dinosaurs would have found plant material scarce, and carnivores would have shortly found prey in short supply.
Several researchers have stated that there were Paleocene dinosaurs and therefore the extinction of dinosaurs was gradual. Their arguments were based on the discovery of dinosaur remains in the Hell Creek Formation up to Template:M to ft above and 40,000 years later than the K–T boundary. In 2001, it was argued by Fassett and colleagues that pollen samples recovered near a fossilized hadrosaur femur recovered in the Ojo Alamo Sandstone at the San Juan River indicate that the animal lived in Tertiary times, approximately 64.5 mya (about 1 million years after the K–T event). This would make hadrosaurids a Dead Clade Walking. Most workers, including Sullivan, contend that these apparent "Paleocene dinosaurs" are "re-worked", that is, the fossils were eroded from their original locations and then re-buried in much later sediments.
Mammals
The Northern hemisphere marsupial families became extinct, but those in Australia and South America survived. Other mammalian families, including monotremes (egg-laying mammals), multituberculates, and placentals, the ancestors of most modern mammals, survived the K–T event. Mammalian species began diversifying approximately 30 million years prior to the K-T boundary; however, further diversification actually stalled across the boundary, which indicates that mammals filled ecological niches during the Cretaceous that were least impacted by the extinction event. A few orders of mammals did diversify right at the K-T boundary, including Chiroptera (bats) and Cetartiodactyla (whales and dolphins and Even-toed ungulates), as a result of the reduced competition in those niches.
K-T boundary mammalian species were relatively small (most less than 1 kg) which allowed them to find shelter in a number of different environments. In addition, many early monotremes and marsupials were semiaquatic or burrowing which also provided protection from K-T boundary environmental stresses.
Evidence
North American fossils

In North American terrestrial sequences, the extinction event is best represented by the marked discrepancy between the rich and relatively abundant late-Maastrichtian palynomorph record and the post-boundary fern spike.
At present the most informative sequence of dinosaur-bearing rocks is found in Montana, USA, comprised of the Hell Creek, Lance and Scollard formations. The fossil beds are found in strata from about 83.5 mya (million years ago) to 64.9 mya, covering the Campanian and Maastrichtian ages of the Cretaceous through the beginning of the Paleocene period. They show changes in dinosaur populations over the last 18 million years of the Cretaceous. Although the Hell Creek, Lance and Scollard formations provide a wealth of information, they cover a relatively small area and it cannot be assumed that these formations represent a worldwide picture of dinosaur life at the end of the Cretaceous.
The middle–late Campanian formations show a greater diversity of dinosaurs than any other single group of rocks. The late Maastrichtian rocks contain the largest members of several major clades: Tyrannosaurus, Ankylosaurus, Pachycephalosaurus, Triceratops and Torosaurus, which suggests prey were plentiful immediately prior to the extinction.
In addition to rich dinosaur fossils, there are also plant fossils that illustrate the reduction in plant species across the K-T boundary. In the sediments below the K–T boundary the dominant plant remains are angiosperm pollen grains, but the actual boundary layer contains little pollen and is dominated by fern spores. Normal pollen levels gradually resume above the boundary layer. This is reminiscent of areas blighted by volcanic eruptions, where the recovery is led by ferns which are later replaced by larger angiosperm plants.
Marine fossils
The mass extinction of marine plankton appears to have been abrupt and right at the K–T boundary. Ammonite genera became extinct at or near the K–T boundary; however, there was a smaller and slower extinction of ammonite genera prior to the boundary that was associated with a late Cretaceous marine regression. The gradual extinction of most inoceramid bivalves began well before the K–T boundary, and a small, gradual reduction in ammonite diversity occurred throughout the very late Cretaceous. Further analysis shows that several processes were in progress in the late Cretaceous seas and partially overlapped in time, then ended with the abrupt mass extinction.
Duration
The length of time taken for the extinction to occur is a controversial issue, because some theories about the extinction's causes require a rapid extinction over a relatively short period (from a few years to a few thousand years) while others require longer periods. The issue is difficult to resolve because of the Signor-Lipps effect; that is, the fossil record is so incomplete that most extinct species probably died out long after the most recent fossil that has been found. Scientists have also found very few continuous beds of fossil-bearing rock which cover a time range from several million years before the K–T extinction to a few million years after it.
Geological cause of extinctions
There have been several theories on cause of the K-T boundary which lead to the massive extinction. These theories have centered on either impact events or increased volcanism; some include elements of both. A scenario combining three major postulated causes: volcanism, marine regression, and extraterrestrial impact has been proposed. In this scenario, terrestrial and marine communities were stressed by the changes in and loss of habitats. Dinosaurs, as the largest vertebrates, were the first to be affected by environmental changes, and their diversity declined. At the same time, particulate materials from volcanism cooled and dried areas of the globe. Then, an impact event occurred, causing collapses in photosynthesis-based food chains, both in the already-stressed terrestrial food chains and in the marine food chains. The major difference between this hypothesis and the single-cause hypotheses is that its proponents view the suggested single causes as either not sufficient in strength to cause the extinctions or not likely to produce the taxonomic pattern of the extinction.
Impact event
Main articles: K-T boundary, Alvarez hypothesis, and Chicxulub Crater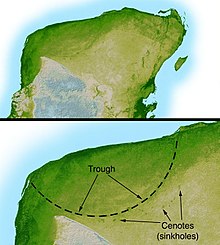
In 1980, a team of researchers consisting of Nobel prize-winning physicist Luis Alvarez, his son geologist Walter Alvarez, and chemists Frank Asaro and Helen Michels discovered that sedimentary layers found all over the world at the Cretaceous–Tertiary boundary contain a concentration of iridium many times greater than normal (30 times and 130 times background in the two sections originally studied). Iridium is extremely rare in the earth's crust because it is a siderophile, and therefore most of it travelled with the iron as it sank into the earth's core during planetary differentiation. As iridium remains abundant in most asteroids and comets, the Alvarez team suggested that an asteroid struck the earth at the time of the K–T boundary. There were other earlier speculations on the possibility of an impact event, but no evidence had been uncovered at that time.
The obvious consequence of an impact would be a dust cloud which would block sunlight and inhibit photosynthesis for a few years. This would account for the extinction of plants and phytoplankton and of organisms dependent on them (including predatory animals as well as herbivores). However, small creatures whose food chains were based on detritus might have still had a reasonable chance of survival. It is estimated that sulfuric acid aerosols were injected into the stratosphere, leading to a 10–20% reduction in sunlight reaching the earth's surface. It would have taken at least ten years for those aerosols to dissipate. The consequences of reentry of ejecta into Earth's atmosphere may have included a brief (hours long) but intense pulse of infrared radiation of an intensity similar to an oven set to broil, killing exposed organisms. Global firestorms may have also resulted as incendiary fragments from the blast fell back to Earth. High O2 levels would have supported intense combustion. The level of atmospheric O2 plummeted in the early Tertiary Period. If widespread fires occurred, they would have increased the CO2 content of the atmosphere and caused a temporary greenhouse effect once the dust cloud settled, and this would have exterminated the most vulnerable survivors of the "long winter".
The impact may also have produced acid rain, depending on what type of rock the asteroid struck. However, recent research suggests this effect was relatively minor. Chemical buffers would have limited the changes, and the survival of animals vulnerable to acid rain effects (such as frogs) indicate this was not a major contributor to extinction. Impact theories can only explain very rapid extinctions, since the dust clouds and possible sulphuric aerosols would wash out of the atmosphere in a fairly short time—possibly under ten years. Subsequent research, however, identified the Chicxulub Crater buried under Chicxulub on the coast of Yucatan, Mexico as the impact crater which matched the Alvarez hypothesis dating. Identified in 1990 based on the work of Glen Penfield done in 1978, this crater is oval, with an average diameter of about Template:Km to mi, about the size calculated by the Alvarez team.
The shape and location of the crater indicate further causes of devastation in addition to the dust cloud. The asteroid landed right on the coast and would have caused gigantic tsunamis, for which evidence has been found all round the coast of the Caribbean and eastern United States—marine sand in locations which were then inland, and vegetation debris and terrestrial rocks in marine sediments dated to the time of the impact. The asteroid landed in a bed of gypsum (calcium sulphate), which would have produced a vast sulphur dioxide aerosol. This would have further reduced the sunlight reaching the earth's surface and then precipitated as acid rain, killing vegetation, plankton and organisms which build shells from calcium carbonate (coccolithophores and molluscs). Most paleontologists now agree that an asteroid did hit the Earth about 65 mya, but there is an ongoing dispute whether the impact was the sole cause of the extinctions.
Deccan Traps
Main article: Deccan TrapsBefore 2000, arguments that the Deccan Traps flood basalts caused the extinction were usually linked to the view that the extinction was gradual, as the flood basalt events were thought to have started around 68 mya and lasted for over 2 million years. However, there is evidence that two-thirds of the Deccan Traps were created in 1 million years about 65.5 mya, so these eruptions would have caused a fairly rapid extinction, possibly a period of thousands of years, but still a longer period than what would be expected from a single impact event.
The Deccan Traps could have caused extinction through several mechanisms, including the release of dust and sulphuric aerosols into the air which might have blocked sunlight and thereby reducing photosynthesis in plants. In addition, Deccan Trap volcanism might have resulted in carbon dioxide emissions which would have increased the greenhouse effect when the dust and aerosols cleared from the atmosphere.
In the years when the Deccan Traps theory was linked to a slower extinction, Luis Alvarez (who died in 1988) replied that paleontologists were being misled by sparse data. While his assertion was not initially well-received, later intensive field studies of fossil beds lent weight to his claim. Eventually, most paleontologists began to accept the idea that the mass extinctions at the end of the Cretaceous were largely or at least partly due to a massive Earth impact. However, even Walter Alvarez has acknowledged that there were other major changes on Earth even before the impact, such as a drop in sea level and massive volcanic eruptions that produced the Indian Deccan Traps, and these may have contributed to the extinctions.
Multiple impact event
Several other craters also appear to have been formed about the time of the K–T boundary. This suggests the possibility of near simultaneous multiple impacts, perhaps from a fragmented asteroidal object, similar to the Shoemaker-Levy 9 cometary impact with Jupiter. Among these are the Boltysh crater, a Template:Km to mi diameter impact crater in the Ukraine (65.17 ± 0.64 mya); the Silverpit crater, a Template:Km to mi diameter impact crater in the North Sea (60–65 mya). Any other craters that might have formed in the Tethys Ocean would have been obscured by tectonic events like the relentless northward drift of Africa and India.
In September 2007, a team made up of scientists from the Southwest Research Institute and the Charles University found evidence of an asteroid breakup of the parent-body of 298 Baptistina, which is conjectured to have occurred about 160 mya. They believe this event may have caused the Chicxulub crater on Earth, and the Tycho crater on the Moon.
Maastrichtian sea-level regression
There is clear evidence that sea levels fell in the final stage of the Cretaceous by more than at any other time in the Mesozoic era. In some Maastrichtian stage rock layers from various parts of the world, the later ones are terrestrial; earlier ones represent shorelines and the earliest represent seabeds. These layers do not show the tilting and distortion associated with mountain building, therefore, the likeliest explanation is a "regression", that is, a drop in sea level. There is no direct evidence for the cause of the regression, but the explanation which is currently accepted as the most likely is that the mid-ocean ridges became less active and therefore sank under their own weight.
A severe regression would have greatly reduced the continental shelf area, which is the most species-rich part of the sea, and therefore could have been enough to cause a marine mass extinction. However research concludes that this change would have been insufficient to cause the observed level of ammonite extinction. The regression would also have caused climate changes, partly by disrupting winds and ocean currents and partly by reducing the earth's albedo and therefore increasing global temperatures.
Marine regression also resulted in the loss of epeiric seas, such as the Western Interior Seaway of North America. The loss of these seas greatly altered habitats, removing coastal plains that ten million years before had been host to diverse communities such as are found in rocks of the Dinosaur Park Formation. Another consequence was an expansion of freshwater environments, since continental runoff now had longer distances to travel before reaching oceans. While this change was favorable to freshwater vertebrates, those that prefer marine environments, such as sharks, suffered.
See also
- Permian–Triassic extinction event – a much larger earlier extinction event
- Tunguska event
Footnotes
- "Tertiary" is no longer recognized as a formal time or rock unit by the International Commission on Stratigraphy, the K-T event is now also called the Cretaceous-Paleogene (or K-Pg) extinction event by some researchers.
- Fortey, R (1999). Life: A Natural History of the First Four Billion Years of Life on Earth. Vintage. pp. 238–260. ISBN 978-0375702617.
- Favstovsky, D.E., and Sheehan, P.M. (2005). "The extinction of the dinosaurs in North America". GSA Today. 15 (3): 4–10. doi:10.1130/1052-5173(2005)015%3C4:TEOTDI%3E2.0.CO;2. Retrieved 2007-05-18.
{{cite journal}}
: CS1 maint: multiple names: authors list (link) - ^ Sloan, R. E., Rigby, K,. Van Valen, L. M., Gabriel, Diane (1986). "Gradual dinosaur extinction and simultaneous ungulate radiation in the Hell Creek formation". Science. 232: 629–633. doi:10.1126/science.232.4750.629. Retrieved 2007-05-18.
{{cite journal}}
: Check|doi=
value (help); Unknown parameter|isue=
ignored (help)CS1 maint: multiple names: authors list (link) - ^ Fassett, JE, Lucas, SG, Zielinski, RA, and Budahn, JR (2001). "Compelling new evidence for Paleocene dinosaurs in the Ojo Alamo Sandstone, San Juan Basin, New Mexico and Colorado, USA" (PDF). Catastrophic events and mass extinctions, Lunar and Planetary Contribution. 1053: 45–46. Retrieved 2007-05-18.
{{cite journal}}
: CS1 maint: multiple names: authors list (link) - ^ Sullivan, RM (2003). "No Paleocene dinosaurs in the San Juan Basin, New Mexico". Geological Society of America Abstracts with Programs. 35 (5): 15. Retrieved 2007-07-02.
- ^ MacLeod, N, Rawson, PF, Forey, PL, Banner, FT, Boudagher-Fadel, MK, Bown, PR, Burnett, JA, Chambers, P, Culver, S, Evans, SE, Jeffery, C, Kaminski, MA, Lord, AR, Milner, AC, Milner, AR, Morris, N, Owen, E, Rosen, BR, Smith, AB, Taylor, PD, Urquhart, E & Young, JR (1997). "The Cretaceous–Tertiary biotic transition". Journal of the Geological Society. 154 (2): 265–292.
{{cite journal}}
: CS1 maint: multiple names: authors list (link) - Wilf, P & Johnson KR (2004). "Land plant extinction at the end of the Cretaceous: a quantitative analysis of the North Dakota megafloral record". Paleobiology. 30 (3): 347–368. doi:10.1666/0094-8373(2004)030%3C0347:LPEATE%3E2.0.CO;2.
- Kauffman, E (2004). "Mosasaur Predation on Upper Cretaceous Nautiloids and Ammonites from the United States Pacific Coast". Palaios. 19 (1). Society for Sedimentary Geology: 96–100. Retrieved 2007-06-17.
{{cite journal}}
: Cite has empty unknown parameter:|coauthors=
(help) - ^ Shehan, P & Hansen, TA (1986). "Detritus feeding as a buffer to extinction at the end of the Cretaceous". Geology. 14 (10): 868–870. Retrieved 2007-07-04.
{{cite journal}}
: CS1 maint: multiple names: authors list (link) - Aberhan, M, Weidemeyer, S, Kieesling, W, Scasso, RA, & Medina, FA (2007). "Faunal evidence for reduced productivity and uncoordinated recovery in Southern Hemisphere Cretaceous-Paleogene boundary sections". Geology. 35 (3): 227–230. doi:10.1130/G23197A.1.
{{cite journal}}
: CS1 maint: multiple names: authors list (link) - Sheehan, PM & Fastovsky, DE (1992). "Major extinctions of land-dwelling vertebrates at the Cretaceous–Tertiary boundary, eastern Montana". Geology. 20 (6): 556–560. Retrieved 2007-06-22.
{{cite journal}}
: CS1 maint: multiple names: authors list (link) - Pospichal, JJ (1996). "Calcareous nannofossils and clastic sediments at the Cretaceous–Tertiary boundary, northeastern Mexico". Geology. 24 (3): 255–258.
- Bown, P (2005). "Selective calcareous nannoplankton survivorship at the Cretaceous–Tertiary boundary". Geology. 33 (8): 653–656. Retrieved 2007-08-01.
- Bambach, R.K.; Knoll, A.H.; Wang, <link rel="stylesheet" type="text/css" href="http://en.wikipedia.org/search/?title=User:Lupin/navpop.css&action=raw&ctype=text/css&dontcountme=s">S.C. (2004). "Origination, extinction, and mass depletions of marine diversity". Paleobiology. 30 (4): 522–542. doi:10.1666/0094-8373(2004)030%3C0522:OEAMDO%3E2.0.CO;2. Retrieved 2007-06-22.
{{cite journal}}
:|first3=
has generic name (help) - Gedl, P (2004). "Dinoflagellate cyst record of the deep-sea Cretaceous-Tertiary boundary at Uzgru, Carpathian Mountains, Czech Republic". Geological Society, London, Special Publications. 230: 257–273. doi:10.1144/GSL.SP.2004.230.01.13.
- MacLeod, N (1998). "Impacts and marine invertebrate extinctions". Geological Society, London, Special Publications. 140: 217–246. Retrieved 2007-08-01.
- Courtillot, V (1999). Evolutionary Catastrophes: The Science of Mass Extinction. Cambridge University Press. p. 2. ISBN 0521583926.
{{cite book}}
: Cite has empty unknown parameter:|coauthors=
(help) - Glaessner, MF (1937). "Studien Ober Foraminiferen aus der Kreide und dem Tertiar des Kaukasus. I-Die Foraminiferen der altesten Tertifrschichten des Nordwestkaukasus". Problems in Paleontology. 2–3: 349–410.
- Arenillas, I, Arz, JA, Molina, E & Dupuis, C (2000). "An Independent Test of Planktic Foraminiferal Turnover across the Cretaceous/Paleogene (K/P) Boundary at El Kef, Tunisia: Catastrophic Mass Extinction and Possible Survivorship". Micropaleontology. 46 (1): 31–49.
{{cite journal}}
: CS1 maint: multiple names: authors list (link) - MacLeod, N (1996). Nature of the Cretaceous-Tertiary (K T) planktonic foraminiferal record: stratigraphic confidence intervals, Signor-Lipps effect, and patterns of survivorship. In: Cretaceous–Tertiary Mass Extinctions: Biotic and Environmental Changes (MacLeod, N. & Keller, G., editors). WW Norton & Company. pp. 85–138. ISBN 978-0393966572.
- ^ Keller, G, Adatte, T, Stinnesbeck, W, Rebolledo-Vieyra, Fucugauchi, JU, Kramar, U, & Stüben, D (2004). "Chicxulub impact predates the K-T boundary mass extinction". PNAS. 101: 3753–3758. doi:10.1073/pnas.0400396101.
{{cite journal}}
: CS1 maint: multiple names: authors list (link) - Galeotti S, Bellagamba M, Kaminski MA, & Montanari A (2002). "Deep-sea benthic foraminiferal recolonisation following a volcaniclastic event in the lower Campanian of the Scaglia Rossa Formation (Umbria^Marche Basin, central Italy)" (PDF). Marine Micropaleontology: 57–76. Retrieved 2007-08-19.
{{cite journal}}
: Unknown parameter|Volume=
ignored (|volume=
suggested) (help)CS1 maint: multiple names: authors list (link) - Kuhnt W & Collins ES (1996). "8. Cretaceous TO Paleogene benthic foramanifers from the Iberia abyssal plain" (PDF). Proceedings of the Ocean Drilling Program, Scientific Results. 149: 203–216. Retrieved 2007-08-19.
- Coles GP, Ayress MA, and Whatley RC (1990). A comparison of North Atlantic and 20 Pacific deep-sea Ostracoda in Ostracoda and global events (RC Whatley and C Maybury, editors). Chapman & Hall. pp. 287–305. ISBN 978-0442311674.
{{cite book}}
: CS1 maint: multiple names: authors list (link) - Brouwers EM & De Deckker P (1993). "Late Maastrichtian and Danian Ostracode Faunas from Northern Alaska: Reconstructions of Environment and Paleogeography". Palaios. 8 (2): 140–154. doi:10.2307/3515168.
- Vescsei, A & Moussavian, E (1997). "Paleocene reefs on the Maiella Platform Margin, Italy: An example of the effects of the cretaceous/tertiary boundary events on reefs and carbonate platforms". Facies. 36 (1): 123–139. doi:10.1007/BF02536880.
{{cite journal}}
: CS1 maint: multiple names: authors list (link) - Rosen, BR & Turnsek, D (1989). "Extinction patterns and biogeography of scleractinian corals across the Cretaceous/Tertiary boundary. Fossil Cnidaria 5.P.". Association of Australasian Paleontology Memoir Number 8, Jell, A & Pickett, JW (eds): 355–370.
{{cite journal}}
: CS1 maint: multiple names: authors list (link) - Ward, PD, Kennedy, WJ, MacLeod, KG, and Mount, JF (1991). "Ammonite and inoceramid bivalve extinction patterns in Cretaceous/Tertiary boundary sections of the Biscay region (southwestern France, northern Spain)". Geology. 19 (12): 1181–1184.
{{cite journal}}
: CS1 maint: multiple names: authors list (link) - Harries PJ, Johnson KR, Cobban WA, Nichols DJ (2002). "Marine Cretaceous-Tertiary boundary section in southwestern South Dakota: Comment and Reply". Geology. 30 (10): 954–955.
{{cite journal}}
: CS1 maint: multiple names: authors list (link) - Neraudeau D, Thierry J & Moreau P (1997). "Variation in echinoid biodiversity during the Cenomanian–early Turonian transgressive episode in Charentes (France)". Bulletin de la Société géologique de France. 168: 51–61.
- Raup DM and Jablonski D (1993). "Geography of end-Cretaceous marine bivalve extinctions". Science. 260 (5110): 971–973. doi:10.1126/science.11537491.
- MacLeod, KG (1994). "Extinction of Inoceramid Bivalves in Maastrichtian Strata of the Bay of Biscay Region of France and Spain". Journal of Paleontology. 68 (5): 1048–1066.
- Patterson, C (1993). Osteichthyes: Teleostei. In: The Fossil Record 2 (Benton, MJ, editor). Springer. pp. 621–656. ISBN 978-0412393808.
- Zinsmeister WJ (1998). "Discovery of fish mortality horizon at the K-T boundary on Seymour Island: Re-evaluation of events at the end of the Cretaceous". Journal of Paleontology. 72 (3): 556–571. Retrieved 2007-08-27.
- ^ Robertson DS, McKenna MC, Toon OB, Hope S & Lillegraven JA (2004). "Survival in the first hours of the Cenozoic" (PDF). GSA Bulletin. 116 (5–6): 760–768. doi:10.1130/B25402.1. Retrieved 2007-08-31.
{{cite journal}}
: CS1 maint: multiple names: authors list (link) - ^ Labandiera, Conrad C. (2002). "Impact of the terminal Cretaceous event onplant–insect associations" (PDF). Proceedings of the National Academy of Sciences of the United States of America. 99 (4): 2061–2066. Retrieved 2007-07-06.
{{cite journal}}
: Unknown parameter|coauthors=
ignored (|author=
suggested) (help) - Wilf, P, Labandeira, CC, Johnson, KR, & Ellis, B (2006). "Decoupled Plant and Insect Diversity After the End-Cretaceous Extinction". Science. 313 (5790): 1112–1115. doi:10.1126/science.1129569.
{{cite journal}}
: CS1 maint: multiple names: authors list (link) - ^ Vajda, Vivi (2001). "Indication of Global Deforestation at the Cretaceous–Tertiary Boundary by New Zealand Fern Spike". Science. 294 (5547): 1700–1702. doi:10.1126/science.1064706. Retrieved 2007-07-05.
{{cite journal}}
: Unknown parameter|coauthors=
ignored (|author=
suggested) (help) - Johnson, KR & Hickey, LJ (1991). Megafloral change across the Cretaceous Tertiary boundary in the northern Great Plains and Rocky Mountains. In: Global Catastrophes in Earth History: An Interdisciplinary Conference on Impacts, Volcanism, and Mass Mortality, Sharpton, VI and Ward, PD (editors). Geological Society of America. ISBN 978-0813722474.
{{cite book}}
: CS1 maint: multiple names: authors list (link) - Askin, RA & Jacobson, SR (1996). Palynological change across the Cretaceous–Tertiary boundary on Seymour Island, Antarctica: environmental and depositional factors. In: Cretaceous–Tertiary Mass Extinctions: Biotic and Environmental Changes, Keller, G & MacLeod, N (editors). WW Norton & Company. ISBN 978-0393966572.
{{cite book}}
: CS1 maint: multiple names: authors list (link) - Schultz, P.H. (1996). "Cretaceous–Tertiary (Chicxulub) impact angle and its consequences". Geology. 24 (11): 963–967. Retrieved 2007-07-05.
{{cite journal}}
: Unknown parameter|coauthors=
ignored (|author=
suggested) (help) - Vajda, V & McLoughlin S (2004). "Fungal Proliferation at the Cretaceous–Tertiary Boundary" (PDF). Science. 303: 1489–1490. doi:10.1126/science.1093807. Retrieved 2007-07-07.
- ^ Archibald, JD & Bryant, LJ (1990). "Differential Cretaceous–Tertiary extinction of nonmarine vertebrates; evidence from northeastern Montana. In: Global Catastrophes in Earth History: an Interdisciplinary Conference on Impacts, Volcanism, and Mass Mortality (Sharpton, VL and Ward, PD, editors)". Geological Society of America, Special Paper. 247: 549–562.
{{cite journal}}
: CS1 maint: multiple names: authors list (link) - ^ Novacek MJ (199). "100 Million Years of Land Vertebrate Evolution: The Cretaceous-Early Tertiary Transition". Annals of the Missouri Botanical Garden. 86 (2): 230–258. doi:10.2307/2666178.
- Lutz D (2005). Tuatara: A Living Fossil. DIMI Press. ISBN 0-931625-43-2.
- Chatterjee S & Small BJ (1989). "New plesiosaurs from the Upper Cretaceous of Antarctica". Geological Society, London, Special Publications. 47: 197–215. Retrieved 2007-07-04.
- O'Keefe FR (2001). "A cladistic analysis and taxonomic revision of the Plesiosauria (Reptilia: Sauropterygia)". Acta Zoologica Fennica. 213: 1–63.
- Brochu CA (2004). "Calibration age and quartet divergence date estimation". Evolution. 58 (6): 1375–1382. doi:10.1554/03-509.
- Slack KE, Jones CM, Ando T, Harrison GL, Fordyce RE, Arnason U & Penny D (2006). "Early Penguin Fossils, Plus Mitochondrial Genomes, Calibrate Avian Evolution". Molecular Biology and Evolution. 23: 1144–1155. Retrieved 2007-08-27.
{{cite journal}}
: CS1 maint: multiple names: authors list (link) - Penny D & Phillips MJ (2004). "The rise of birds and mammals: are microevolutionary processes sufficient for macroevolution". Trends Ecol. Evol. 19: 516–522.
- Hou L, Martin M, Zhou Z & Feduccia A (1996). "Early Adaptive Radiation of Birds: Evidence from Fossils from Northeastern China". Science. 274 (5290): 1164–1167. doi:10.1126/science.274.5290.1164.
{{cite journal}}
: CS1 maint: multiple names: authors list (link) - Clarke JA, Tambussi CP, Noriega JI, Erickson GM, & Ketcham RA (2005). "Definitive fossil evidence for the extant avian radiation in the Cretaceous". Nature. 433 (7023): 305–308. doi:10.1038/nature03150.
{{cite journal}}
: CS1 maint: multiple names: authors list (link) - ^ Archibald, J. David (2004). "Dinosaur Extinction". In Weishampel, David B.; Dodson, Peter; and Osmólska, Halszka (eds.) (ed.). The Dinosauria (2nd ed.). Berkeley: University of California Press. pp. 672–684. ISBN 0-520-24209-2.
{{cite book}}
:|editor=
has generic name (help); Unknown parameter|coauthors=
ignored (|author=
suggested) (help)CS1 maint: multiple names: editors list (link) - ^ Dodson, P. (1996). The Horned Dinosaurs: A Natural History. Princeton: Princeton University Press. pp. 279–281. ISBN 0-691-05900-4.
- Bininda-Emonds, ORP, Cardillo, M, Jones, KE, MacPhee, RDE, Beck, RMD, Grenyer, R, Price, SA, Vos, RA, Gittleman, JL Y Purvis, A (2007). "The delayed rise of present-day mammals". Nature. 446: 507–512. doi:10.1038/nature05634.
{{cite journal}}
: CS1 maint: multiple names: authors list (link) - Springer, MS, Murphy, WJ, Eizirik, E, & O'Brien, SJ (2003). "Placental mammal diversification and the Cretaceous–Tertiary boundary" (PDF). PNAS. 100 (3): 1056–1061. doi:10.1073/pnas.0334222100. Retrieved 2007-07-20.
{{cite journal}}
: CS1 maint: multiple names: authors list (link) - Gelfo JN & Pascual R (2001). "Peligrotherium tropicalis (Mammalia, Dryolestida) from the early Paleocene of Patagonia, a survival from a Mesozoic Gondwanan radiation". Geodiversitas. 23: 369–379.
- "Online guide to the continental Cretaceous–Tertiary boundary in the Raton basin, Colorado and New Mexico". U.S. Geological Survey. 2004. Retrieved 2007-07-08.
- Smathers, GA & Mueller-Dombois, D (1974). Invasion and Recovery of Vegetation after a Volcanic Eruption in Hawai'i, Scientific Monograph Number 5. United States National Park Service. Retrieved 2007-07-09.
{{cite book}}
: CS1 maint: multiple names: authors list (link) - ^ Pope, KO, D'Hondt, SL & Marshall, CR (1998). "Meteorite impact and the mass extinction of species at the Cretaceous/Tertiary boundary". PNAS. 95 (19): 11028–11029. PMID 9736679. Retrieved 2007-06-15.
{{cite journal}}
: CS1 maint: multiple names: authors list (link) - ^ Marshall, C. R. & Ward, PD (1996). "Sudden and Gradual Molluscan Extinctions in the Latest Cretaceous of Western European Tethys". Science. 274 (5291): 1360–1363. doi:10.1126/science.274.5291.1360.
{{cite journal}}
: CS1 maint: multiple names: authors list (link) - Signor III, P. W. and Lipps, J. H. (1982). "Sampling bias, gradual extinction patterns, and catastrophes in the fossil record. In: Geological implications of impacts of large asteroids and comets on the Earth, Silver, LT & Schultz, PH (editors)". Geological Society of America Special Publication. 190: 291–296.
{{cite journal}}
: CS1 maint: multiple names: authors list (link) - Alvarez, LW, Alvarez, W, Asaro, F, and Michel, HV (1980). "Extraterrestrial cause for the Cretaceous–Tertiary extinction". Science. 208 (4448): 1095–1108. doi:10.1126/science.208.4448.1095.
{{cite journal}}
: CS1 maint: multiple names: authors list (link) - De Laubenfels, MW (1956). "Dinosaur Extinctions: One More Hypothesis". Journal of Paleontology. 30 (1): 207–218. Retrieved 2007-05-22.
- Ocampo, A, Vajda, V & Buffetaut, E (2006). Unravelling the Cretaceous–Paleogene (KT) Turnover, Evidence from Flora, Fauna and Geology in Biological Processes Associated with Impact Events (Cockell, C, Gilmour, I & Koeberl, C, editors). SpringerLink. pp. 197–219. ISBN 978-3-540-25735-6. Retrieved 2007-06-17.
{{cite book}}
: CS1 maint: multiple names: authors list (link) - Pope, KO, Baines, KH, Ocampo, AC, & Ivanov, BA (1997). "Energy, volatile production, and climatic effects of the Chicxulub Cretaceous/Tertiary impact". Journal of Geophysical Research. 102 (E9): 21645–21664. Retrieved 2007-07-18.
{{cite journal}}
: CS1 maint: multiple names: authors list (link) - Kring, DA (2003). "Environmental consequences of impact cratering events as a function of ambient conditions on Earth". Astrobiology. 3 (1): 133–152. PMID 12809133.
- Pope KO, Ocampo AC, Kinsland GL, Smith R (1996). "Surface expression of the Chicxulub crater". Geology. 24 (6): 527–30. PMID 11539331.
{{cite journal}}
: CS1 maint: multiple names: authors list (link) - Morgan, J, Lana, C, Kersley, A, Coles, B, Belcher, C, Montanari, S, Diaz-Martinez, E, Barbosa, A & Neumann, V (2006). "Analyses of shocked quartz at the global K-P boundary indicate an origin from a single, high-angle, oblique impact at Chicxulub". Earth and Planetary Science Letters. 251 (3–4): 264–279. doi:10.1016/j.epsl.2006.09.009.
{{cite journal}}
: CS1 maint: multiple names: authors list (link) - Hofman, C, Féraud, G & Courtillot, V (2000). "40Ar/39Ar dating of mineral separates and whole rocks from the Western Ghats lava pile: further constraints on duration and age of the Deccan traps". Earth and Planetary Science Letters. 180: 13–27. doi:10.1016/S0012-821X(00)00159-X.
{{cite journal}}
: CS1 maint: multiple names: authors list (link) - ^ Duncan, RA & Pyle, DG (1988). "Rapid eruption of the Deccan flood basalts at the Cretaceous/Tertiary boundary". Nature. 333: 841–843. doi:10.1038/333841a0.
{{cite journal}}
: CS1 maint: multiple names: authors list (link) - Alvarez, W (1997). T. rex and the Crater of Doom. Princeton University Press. pp. 130–146. ISBN 978-0691016306.
- Mullen, L (October 13 2004). "Debating the Dinosaur Extinction". Astrobiology Magazine. Retrieved 2007-07-11.
{{cite journal}}
: Check date values in:|date=
(help) - Mullen, L (October 20, 2004). "Multiple impacts". Astrobiology Magazine. Retrieved 2007-07-11.
{{cite journal}}
: Check date values in:|date=
(help) - Mullen, L (November 03 2004). "Shiva: Another K–T impact?". Astrobiology Magazine. Retrieved 2007-07-11.
{{cite journal}}
: Check date values in:|date=
(help) - Liangquan, L (1998). "Abrupt deep-sea warming at the end of the Cretaceous". Geology. 26: 995–998. Retrieved 2007-08-01.
{{cite journal}}
: Unknown parameter|coauthors=
ignored (|author=
suggested) (help)
Further reading
- Fortey, R (2005). Earth: An Intimate History. Vintage. ISBN 978-0375706202.
External links
- "The Chicxulub Debate". Princeton University. 2007. Retrieved 2007-08-02.
- McLean, D (1995). "The Deccan Traps Volcanism-Greenhouse Dinosaur Extinction Theory". University of Vermont. Retrieved 2007-08-02.
- Kring, DA (2005). "Chicxulub Impact Event: Understanding the K–T Boundary". NASA Space Imagery Center. Retrieved 2007-08-02.
- Cowen, R (2000). "The K–T extinction". University of California Museum of Paleontology. Retrieved 2007-08-02.
- "What killed the dinosaurs?". University of California Museum of Paleontology. Retrieved 2007-08-02.
{{cite web}}
: Text "date-1995" ignored (help) - Rincon, P (2004-03-01). "Dinosaur impact theory challenged". BBC News. Retrieved 2007-08-02.
{{cite web}}
: Check date values in:|date=
(help) - Lovett, RA (2006-10-30). ""Dinosaur Killer" Asteroid Only One Part of New Quadruple-Whammy Theory". National Geographic News. Retrieved 2007-08-02.
{{cite web}}
: Check date values in:|date=
(help)
Cretaceous–Paleogene extinction event | |
---|---|
Proposed Alvarez hypothesis craters |
Extinction events | |
---|---|
Minor events↓End-Ediacaran?↓Lau event↓Toarcian turnover↓Aptian↓Cenomanian-Turonian↓Middle Miocene↓Rainforest collapse↓Capitanian↓Smithian-Spathian↓Cambrian-Ordovician↓Olson's↓Ordovician-Silurian↓Late Devonian↓Permo-Triassic↓Triassic–Jurassic↓Cretaceous–Paleogene↓Holocene Major eventsEdiacaranCambrianOrdovicianSilurianDevonianCarboniferousPermianTriassicJurassicCretaceousPaleogeneNeogeneQuaternaryNeoproterozoicPalæozoicMesozoicCenozoic│−600│−550│−500│−450│−400│−350│−300│−250│−200│−150│−100│−50│0Millions of years before present |
Categories: