Revision as of 04:22, 16 March 2011 view source202.45.119.15 (talk) →Effects← Previous edit | Revision as of 04:24, 16 March 2011 view source 202.45.119.15 (talk) →GravityNext edit → | ||
Line 41: | Line 41: | ||
==Processes== | ==Processes== | ||
===Gravity=== | ===Gravity=== | ||
] | ]hi im from scotland | ||
'']'' is the down-slope movement of rock and sediments, mainly due to the force of ]. Mass movement is an important part of the erosional process, as it moves material from higher elevations to lower elevations where other eroding agents such as streams and ]s can then pick up the material and move it to even lower elevations. Mass-movement processes are always occurring continuously on all slopes; some mass-movement processes act very slowly; others occur very suddenly, often with disastrous results. Any perceptible down-slope movement of rock or sediment is often referred to in general terms as a ]. However, landslides can be classified in a much more detailed way that reflects the mechanisms responsible for the movement and the velocity at which the movement occurs. One of the visible topographical manifestations of a very slow form of such activity is a ] slope. | '']'' is the down-slope movement of rock and sediments, mainly due to the force of ]. Mass movement is an important part of the erosional process, as it moves material from higher elevations to lower elevations where other eroding agents such as streams and ]s can then pick up the material and move it to even lower elevations. Mass-movement processes are always occurring continuously on all slopes; some mass-movement processes act very slowly; others occur very suddenly, often with disastrous results. Any perceptible down-slope movement of rock or sediment is often referred to in general terms as a ]. However, landslides can be classified in a much more detailed way that reflects the mechanisms responsible for the movement and the velocity at which the movement occurs. One of the visible topographical manifestations of a very slow form of such activity is a ] slope. | ||
Revision as of 04:24, 16 March 2011
For morphological image processing operations, see Erosion (morphology). For use of in dematopathology, see Erosion (dermatopathology).![]() | This article needs additional citations for verification. Please help improve this article by adding citations to reliable sources. Unsourced material may be challenged and removed. Find sources: "Erosion" – news · newspapers · books · scholar · JSTOR (June 2009) (Learn how and when to remove this message) |

Erosion is the process of weathering and transport of solids (sediment, soil, rock and other particles) in the natural environment or their source and deposits them elsewhere. It usually occurs due to transport by wind, water, or ice; by down-slope creep of soil and other material under the force of gravity; or by living organisms, such as burrowing animals, in the case of bioerosion.
Erosion is a natural process, but it has been increased dramatically by human land use, especially industrial agriculture, deforestation, and urban sprawl. Land that is used for industrial agriculture generally experiences a significantly greater rate of erosion than that of land under natural vegetation, or land used for sustainable agricultural practices. This is particularly true if tillage is used, which reduces vegetation cover on the surface of the soil and disturbs both soil structure and plant roots that would otherwise hold the soil in place. However, improved land use practices can limit erosion, using techniques such as terrace-building, conservation tillage practices, and tree planting.
A certain amount of erosion is natural and, in fact, healthy for the ecosystem. For example, gravels continuously move downstream in watercourses. Excessive erosion, however, causes serious problems, such as receiving water sedimentation, ecosystem damage and outright loss of soil.
Erosion is distinguished from weathering, which is the process of chemical or physical breakdown of the minerals in the rocks, although the two processes may occur concurrently.
Causes

The rate of erosion depends on many factors. Climatic factors include the amount and intensity of precipitation, the average temperature, as well as the typical temperature range, and seasonality, the wind speed, storm frequency. The geologic factors include the sediment or rock type, its porosity and permeability, the slope (gradient) of the land, and whether the rocks are tilted, faulted, folded, or weathered. The biological factors include ground cover from vegetation or lack thereof, the type of organisms inhabiting the area, and the land use.
In general, given similar vegetation and ecosystems, areas with high-intensity precipitation, more frequent rainfall, more wind, or more storms are expected to have more erosion. Sediment with high sand or silt contents and areas with steep slopes erode more easily, as do areas with highly fractured or weathered rock. Porosity and permeability of the sediment or rock affect the speed with which the water can percolate into the ground. If the water moves underground, less runoff is generated, reducing the amount of surface erosion. Sediments containing more clay tend to erode less than those with sand or silt. Here, however, the impact of atmospheric sodium on erodibility of clay should be considered.
The factor that is most subject to change is the amount and type of ground cover. In an undisturbed forest, the mineral soil is protected by a litter layer and an organic layer. These two layers protect the soil by absorbing the impact of rain drops. These layers and the underlying soil in a forest are porous and highly permeable to rainfall. Typically, only the most severe rainfall and large hailstorm events will lead to overland flow in a forest. If the trees are removed by fire or logging, infiltration rates become high and erosion low to the degree the forest floor remains intact. Severe fires can lead to significantly increased erosion if followed by heavy rainfall. In the case of construction or road building, when the litter layer is removed or compacted, the susceptibility of the soil to erosion is greatly increased.
Roads are especially likely to cause increased rates of erosion because, in addition to removing ground cover, they can significantly change drainage patterns, especially if an embankment has been made to support the road. A road that has a lot of rock and one that is "hydrologically invisible" (that gets the water off the road as quickly as possible, mimicking natural drainage patterns) has the best chance of not causing increased erosion.
Many human activities remove vegetation from an area, making the soil susceptible to erosion. Logging can cause increased erosion rates due to soil compaction, exposure of mineral soil, for example roads and landings. However it is the removal of or compromise to the forest floor not the removal of the canopy that can lead to erosion. This is because rain drops striking tree leaves coalesce with other rain drops creating larger drops. When these larger drops fall (called throughfall) they again may reach terminal velocity and strike the ground with more energy then had they fallen in the open. Terminal velocity of rain drops is reached in about 8 meters. Because forest canopies are usually higher than this, leaf drop can regain terminal velocity. However, the intact forest floor, with its layers of leaf litter and organic matter, absorbs the impact of the rainfall.
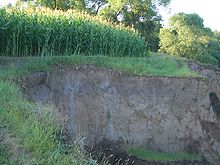
Heavy grazing can reduce vegetation enough to increase erosion. Changes in the kind of vegetation in an area can also affect erosion rates. Different kinds of vegetation lead to different infiltration rates of rain into the soil. Forested areas have higher infiltration rates, so precipitation will result in less surface runoff, which erodes. Instead much of the water will go in subsurface flows, which are generally less erosive. Leaf litter and low shrubs are an important part of the high infiltration rates of forested systems, the removal of which can increase erosion rates. Leaf litter also shelters the soil from the impact of falling raindrops, which is a significant agent of erosion. Vegetation can also change the speed of surface runoff flows, so grasses and shrubs can also be instrumental in this aspect.
One of the main causes of erosive soil loss in the year 2006 is the result of slash and burn treatment of tropical forest. When the total ground surface is stripped of vegetation and then seared of all living organisms, the upper soils are vulnerable to both wind and water erosion. In a number of regions of the earth, entire sectors of a country have been rendered unproductive. For example, on the Madagascar high central plateau, comprising approximately ten percent of that country's land area, virtually the entire landscape is sterile of vegetation, with gully erosive furrows typically in excess of 50 meters deep and one kilometer wide. Shifting cultivation is a farming system which sometimes incorporates the slash and burn method in some regions of the world. This degrades the soil and causes the soil to become less and less fertile.
Effects
Approximately 40% of the world's agricultural land is seriously degraded. According to the UN, an area of fertile soil the size of Ukraine is lost every year because of drought, deforestation and climate change. In Africa, if current trends of soil degradation continue, the continent might be able to feed just 25% of its population by 2025, according to UNU's Ghana-based Institute for Natural Resources in Africa.
When land is overused by animal activities (including humans), there can be mechanical erosion and also removal of vegetation leading to erosion. In the case of the animal kingdom, this effect would become material primarily with very large animal herds stampeding such as the Blue Wildebeest on the Serengeti plain. Even in this case there are broader material benefits to the ecosystem, such as continuing the survival of grasslands, that are indigenous to this region. This effect may be viewed as anomalous or a problem only when there is a significant imbalance or overpopulation of one species.
In the case of human use, the effects are also generally linked to overpopulation. When large number of hikers use trails or extensive off road vehicle use occurs, erosive effects often follow, arising from vegetation removal and furrowing of foot traffic and off road vehicle tires. These effects can also accumulate from a variety of outdoor human activities, again simply arising from too many people using a finite land resource.
One of the most serious and long-running water erosion problems worldwide is in the People's Republic of China, on the middle reaches of the Yellow River and the upper reaches of the Yangtze River. From the Yellow River, over 1.6 billion tons of sediment flows into the ocean each year. The sediment originates primarily from water erosion in the Loess Plateau region of the northwest.
Processes
Gravity
hi im from scotland
Mass wasting is the down-slope movement of rock and sediments, mainly due to the force of gravity. Mass movement is an important part of the erosional process, as it moves material from higher elevations to lower elevations where other eroding agents such as streams and glaciers can then pick up the material and move it to even lower elevations. Mass-movement processes are always occurring continuously on all slopes; some mass-movement processes act very slowly; others occur very suddenly, often with disastrous results. Any perceptible down-slope movement of rock or sediment is often referred to in general terms as a landslide. However, landslides can be classified in a much more detailed way that reflects the mechanisms responsible for the movement and the velocity at which the movement occurs. One of the visible topographical manifestations of a very slow form of such activity is a scree slope.
Slumping happens on steep hillsides, occurring along distinct fracture zones, often within materials like clay that, once released, may move quite rapidly downhill. They will often show a spoon-shaped isostatic depression, in which the material has begun to slide downhill. In some cases, the slump is caused by water beneath the slope weakening it. In many cases it is simply the result of poor engineering along highways where it is a regular occurrence.
Surface creep is the slow movement of soil and rock debris by gravity which is usually not perceptible except through extended observation. However, the term can also describe the rolling of dislodged soil particles 0.5 to 1.0 mm in diameter by wind along the soil surface.
Water

Splash erosion is the detachment and airborne movement of small soil particles caused by the impact of raindrops on soil.
Sheet erosion is the detachment of soil particles by raindrop impact and their removal downslope by water flowing overland as a sheet instead of in definite channels or rills. The impact of the raindrop breaks apart the soil aggregate. Particles of clay, silt and sand fill the soil pores and reduce infiltration. After the surface pores are filled with sand, silt or clay, overland surface flow of water begins due to the lowering of infiltration rates. Once the rate of falling rain is faster than infiltration, runoff takes place. There are two stages of sheet erosion. The first is rain splash, in which soil particles are knocked into the air by raindrop impact. In the second stage, the loose particles are moved downslope by broad sheets of rapidly flowing water filled with sediment known as sheetfloods. This stage of sheet erosion is generally produced by cloudbursts, sheetfloods commonly travel short distances and last only for a short time.
Rill erosion refers to the development of small, ephemeral concentrated flow paths, which function as both sediment source and sediment delivery systems for erosion on hillslopes. Generally, where water erosion rates on disturbed upland areas are greatest, rills are active. Flow depths in rills are typically on the order of a few centimeters or less and slopes may be quite steep. These conditions constitute a very different hydraulic environment than typically found in channels of streams and rivers. Eroding rills evolve morphologically in time and space. The rill bed surface changes as soil erodes, which in turn alters the hydraulics of the flow. The hydraulics is the driving mechanism for the erosion process, and therefore dynamically changing hydraulic patterns cause continually changing erosional patterns in the rill. Thus, the process of rill evolution involves a feedback loop between flow detachment, hydraulics, and bed form. Flow velocity, depth, width, hydraulic roughness, local bed slope, friction slope, and detachment rate are time and space variable functions of the rill evolutionary process. Superimposed on these interactive processes, the sediment load, or amount of sediment in the flow, has a large influence on soil detachment rates in rills. As sediment load increases, the ability of the flowing water to detach more sediment decreases.
Where precipitation rates exceed soil infiltration rates, runoff occurs. Surface runoff turbulence can often cause more erosion than the initial raindrop impact.
Gully erosion, also called ephemeral gully erosion, occurs when water flows in narrow channels during or immediately after heavy rains or melting snow. This is particularly noticeable in the formation of hollow ways, where, prior to being tarmacked, an old rural road has over many years become significantly lower than the surrounding fields.
A gully is sufficiently deep that it would not be routinely destroyed by tillage operations, whereas rill erosion is smoothed by ordinary farm tillage. The narrow channels, or gullies, may be of considerable depth, ranging from 1 to 2 feet (0.61 m) to as much as 75 to 100 feet (30 m). Gully erosion is not accounted for in the revised universal soil loss equation.
Valley or stream erosion occurs with continued water flow along a linear feature. The erosion is both downward, deepening the valley, and headward, extending the valley into the hillside. In the earliest stage of stream erosion, the erosive activity is dominantly vertical, the valleys have a typical V cross-section and the stream gradient is relatively steep. When some base level is reached, the erosive activity switches to lateral erosion, which widens the valley floor and creates a narrow floodplain. The stream gradient becomes nearly flat, and lateral deposition of sediments becomes important as the stream meanders across the valley floor. In all stages of stream erosion, by far the most erosion occurs during times of flood, when more and faster-moving water is available to carry a larger sediment load. In such processes, it is not the water alone that erodes: suspended abrasive particles, pebbles and boulders can also act erosively as they traverse a surface.
At extremely high flows, kolks, or vortices are formed by large volumes of rapidly rushing water. Kolks cause extreme local erosion, plucking bedrock and creating pothole-type geographical features called Rock-cut basins. Examples can be seen in the flood regions result from glacial Lake Missoula, which created the channeled scablands in the Columbia Basin region of eastern Washington.
Bank erosion is the wearing away of the banks of a stream or river. This is distinguished from changes on the bed of the watercourse, which is referred to as scour. Erosion and changes in the form of river banks may be measured by inserting metal rods into the bank and marking the position of the bank surface along the rods at different times.
Shoreline
Main article: Coastal erosion See also: Beach evolution
Shoreline erosion, which occurs on both exposed and sheltered coasts, primarily occurs through the action of currents and waves but sea level (tidal) change can also play a role.
Hydraulic action takes place when air in a joint is suddenly compressed by a wave closing the entrance of the joint. This then cracks it. Wave pounding is when the sheer energy of the wave hitting the cliff or rock breaks pieces off. Abrasion or corrasion is caused by waves launching seaload at the cliff. It is the most effective and rapid form of shoreline erosion (not to be confused with corrosion). Corrosion is the dissolving of rock by carbonic acid in sea water. Limestone cliffs are particularly vulnerable to this kind of erosion. Attrition is where particles/seaload carried by the waves are worn down as they hit each other and the cliffs. This then makes the material easier to wash away. The material ends up as shingle and sand. Another significant source of erosion, particularly on carbonate coastlines, is the boring, scraping and grinding of organisms, a process termed bioerosion.
Sediment is transported along the coast in the direction of the prevailing current (longshore drift). When the upcurrent amount of sediment is less than the amount being carried away, erosion occurs. When the upcurrent amount of sediment is greater, sand or gravel banks will tend to form. These banks may slowly migrate along the coast in the direction of the longshore drift, alternately protecting and exposing parts of the coastline. Where there is a bend in the coastline, quite often a build up of eroded material occurs forming a long narrow bank (a spit). Armoured beaches and submerged offshore sandbanks may also protect parts of a coastline from erosion. Over the years, as the shoals gradually shift, the erosion may be redirected to attack different parts of the shore.
Ice
Ice erosion can take one of two forms. It can be caused by the movement of ice, typically as glaciers, in a process called glacial erosion. It can also be due to freeze-thaw processes in which water inside pores and fractures in rock may expand causing further cracking.
Glaciers erode predominantly by three different processes: abrasion/scouring, plucking, and ice thrusting. In an abrasion process, debris in the basal ice scrapes along the bed, polishing and gouging the underlying rocks, similar to sandpaper on wood. Glaciers can also cause pieces of bedrock to crack off in the process of plucking. In ice thrusting, the glacier freezes to its bed, then as it surges forward, it moves large sheets of frozen sediment at the base along with the glacier. This method produced some of the many thousands of lake basins that dot the edge of the Canadian Shield. These processes, combined with erosion and transport by the water network beneath the glacier, leave moraines, drumlins, ground moraine (till), kames, kame deltas, moulins, and glacial erratics in their wake, typically at the terminus or during glacier retreat.
Cold weather causes water trapped in tiny rock cracks to freeze and expand, breaking the rock into several pieces. This can lead to gravity erosion on steep slopes. The scree which forms at the bottom of a steep mountainside is mostly formed from pieces of rock (soil) broken away by this means. It is a common engineering problem wherever rock cliffs are alongside roads, because morning thaws can drop hazardous rock pieces onto the road.
In some places, water seeps into rocks during the daytime, then freezes at night. Ice expands, thus, creating a wedge in the rock. Over time, the repetition in the forming and melting of the ice causes fissures, which eventually breaks the rock down.
Wind

In arid climates, the main source of erosion is wind. The general wind circulation moves small particulates such as dust across wide oceans thousands of kilometers downwind of their point of origin, which is known as deflation. Erosion can be the result of material movement by the wind. There are two main effects. First, wind causes small particles to be lifted and therefore moved to another region. This is called deflation. Second, these suspended particles may impact on solid objects causing erosion by abrasion (ecological succession). Wind erosion generally occurs in areas with little or no vegetation, often in areas where there is insufficient rainfall to support vegetation. An example is the formation of sand dunes, on a beach or in a desert. Loess is a homogeneous, typically nonstratified, porous, friable, slightly coherent, often calcareous, fine-grained, silty, pale yellow or buff, windblown (aeolian) sediment. It generally occurs as a widespread blanket deposit that covers areas of hundreds of square kilometers and tens of meters thick. Loess often stands in either steep or vertical faces. Loess tends to develop into highly rich soils. Under appropriate climatic conditions, areas with loess are among the most agriculturally productive in the world. Loess deposits are geologically unstable by nature, and will erode very readily. Therefore, windbreaks (such as big trees and bushes) are often planted by farmers to reduce the wind erosion of loess.
Thermal
Thermal erosion is the result of melting and weakening permafrost due to moving water. It can occur both along rivers and at the coast. Rapid river channel migration observed in the Lena River of Siberia is due to thermal erosion, as these portions of the banks are composed of permafrost-cemented non-cohesive materials. Much of this erosion occurs as the weakened banks fail in large slumps. Thermal erosion also affects the Arctic coast, where wave action and near-shore temperatures combine to undercut permafrost bluffs along the shoreline and cause them to fail. Annual erosion rates along a 100-kilometer segment of the Beaufort Sea shoreline averaged 5.6 meters per year from 1955 to 2002.
Soil erosion and climate change
Main article: Land degradationThe warmer atmospheric temperatures observed over the past decades are expected to lead to a more vigorous hydrological cycle, including more extreme rainfall events. In 1998 Karl and Knight reported that from 1910 to 1996 total precipitation over the contiguous U.S. increased, and that 53% of the increase came from the upper 10% of precipitation events (the most intense precipitation). The percent of precipitation coming from days of precipitation in excess of 50 mm has also increased significantly.
Studies on soil erosion suggest that increased rainfall amounts and intensities will lead to greater rates of erosion. Thus, if rainfall amounts and intensities increase in many parts of the world as expected, erosion will also increase, unless amelioration measures are taken. Soil erosion rates are expected to change in response to changes in climate for a variety of reasons. The most direct is the change in the erosive power of rainfall. Other reasons include: a) changes in plant canopy caused by shifts in plant biomass production associated with moisture regime; b) changes in litter cover on the ground caused by changes in both plant residue decomposition rates driven by temperature and moisture dependent soil microbial activity as well as plant biomass production rates; c) changes in soil moisture due to shifting precipitation regimes and evapo-transpiration rates, which changes infiltration and runoff ratios; d) soil erodibility changes due to decrease in soil organic matter concentrations in soils that lead to a soil structure that is more susceptible to erosion and increased runoff due to increased soil surface sealing and crusting; e) a shift of winter precipitation from non-erosive snow to erosive rainfall due to increasing winter temperatures; f) melting of permafrost, which induces an erodible soil state from a previously non-erodible one; and g) shifts in land use made necessary to accommodate new climatic regimes.
Studies by Pruski and Nearing indicated that, other factors such as land use not considered, we can expect approximately a 1.7% change in soil erosion for each 1% change in total precipitation under climate change.
Tectonic effects

The removal by erosion of large amounts of rock from a particular region, and its deposition elsewhere, can result in a lightening of the load on the lower crust and mantle. This can cause tectonic or isostatic uplift in the region. Research undertaken since the early 1990s suggests that the spatial distribution of erosion at the surface of an orogen can exert a key influence on its growth and its final internal structure (see erosion and tectonics).
Materials science
In materials science, erosion is the recession of surfaces by repeated localized mechanical trauma as, for example, by suspended abrasive particles within a moving fluid. Erosion can also occur from non-abrasive fluid mixtures. Cavitation is one example.
In hard particle erosion, the hardness of the impacted material is a large factor in the mechanics of the erosion. A soft material will typically erode fastest from glancing impacts. Harder material will typically erode fastest from perpendicular impacts. Hardness is a correlative factor for erosion resistance, but a higher hardness does not guarantee better resistance. Factors that affect the erosion rate also include impacting particle speed, size, density, hardness, and rotation. Coatings can be applied to retard erosion, but normally can only slow the removal of material. Erosion rate for solid particle impact is typically measured as mass of material removed divided by the mass of impacting material.
Figurative use
The concept of erosion is commonly employed by analogy to various forms of perceived or real homogenization (i.e. erosion of boundaries), "leveling out", collusion or even the decline of anything from morals to indigenous cultures. It is a common trope of the English language to describe as erosion the gradual, organic mutation of something thought of as distinct, more complex, harder to pronounce or more refined into something indistinct, less complex, easier to pronounce or (disparagingly) less refined.
Origin of term
![]() | This section needs additional citations for verification. Please help improve this article by adding citations to reliable sources in this section. Unsourced material may be challenged and removed. (June 2009) (Learn how and when to remove this message) |
The first known occurrence of the term "erosion" was in the 1541 translation by Robert Copland of Guy de Chauliac's medical text The Questyonary of Cyrurygens. Copland used erosion to describe how ulcers developed in the mouth. By 1774 'erosion' was used outside medical subjects. Oliver Goldsmith employed the term in the more contemporary geological context, in his book Natural History, with the quote
- "Bounds are thus put to the erosion of the earth by water."
See also
- Badland
- Bioerosion
- Biorhexistasy
- Bridge scour
- Cellular confinement
- Certified Professional in Erosion and Sediment Control CPESC
- Deforestation
- Desertification
- Erosion control
- Erosion prediction
- Food security
- Geography
- Groundwater sapping
- Highly erodible land
- Lessivage
- Riparian strips
- Sediment transport
- Soft engineering
- Sphericity scale
- Surface runoff
- TERON (Tillage erosion)
- Vegetation and slope stability
- Vetiver System
- Ocean surface wave
Notes
- Montgomery, David (October 2, 2008). Dirt: The Erosion of Civilizations (1st ed.). University of California Press. ISBN 978-0-520-25806-8.
- Kötke, William (November 30, 2007). The Final Empire. AuthorHouse. ISBN 978-1-4343-3130-4.
- Schmittner Karl-Erich and Pierre, 1999. The impact of atmospheric sodium on erodibility of clay in a coastal Mediterranean region. Environmental Geology 37/3: 195-206.
- Concepts about forests and water Author: Stuart, Gordon W.; Edwards, Pamela J.
- Global food crisis looms as climate change and population growth strip fertile land
- I AM FROM SCOTLAND2008: The year of global food crisis
- Africa may be able to feed only 25% of its population by 2025
- Alt, David (2001). Glacial Lake Missoula & its Humongous Floods. Mountain Press Publishing Company. ISBN 978-0-87842-415-3.
- Nancy D. Gordon (2004-06-01). "Erosion and Scour". Stream hydrology: an introduction for ecologists. ISBN 978-0-470-84357-4.
- ^ Vern Hofman and Dave Franzen (1997). "Emergency Tillage to Control Wind Erosion". North Dakota State University Extension Service. Archived from the original on 2010-11-18. Retrieved 2009-03-21.
{{cite web}}
: Unknown parameter|deadurl=
ignored (|url-status=
suggested) (help) - James K. B. Bishop, Russ E. Davis, and Jeffrey T. Sherman (2002). "Robotic Observations of Dust Storm Enhancement of Carbon Biomass in the North Pacific". Science 298. pp. 817–821. Archived from the original on 2010-11-18. Retrieved 2009-06-20.
{{cite web}}
: Unknown parameter|deadurl=
ignored (|url-status=
suggested) (help)CS1 maint: multiple names: authors list (link) - United States Geological Survey (2004). "Dunes – Getting Started". Archived from the original on 2010-11-18. Retrieved 2009-03-21.
{{cite web}}
: Unknown parameter|deadurl=
ignored (|url-status=
suggested) (help) - F. von Richthofen (1882). On the mode of origin of the loess. pp. 293–305.
{{cite book}}
:|work=
ignored (help) - Glossary of Geology. Springer-Verlag, New York. 2005. p. 779. ISBN 978-3-540-27951-8.
{{cite book}}
: Unknown parameter|coauthors=
ignored (|author=
suggested) (help) - Introduction to Geography, Seventh Edition. McGraw Hill. 2000. p. 99. ISBN 978-0-697-38506-2.
{{cite book}}
: Unknown parameter|coauthors=
ignored (|author=
suggested) (help); Unknown parameter|name=
ignored (help) - "Thermal Erosion". NSIDC Glossary. National Snow and Ice Data Center. Archived from the original on 2010-11-18. Retrieved 21 December 2009.
{{cite web}}
: Unknown parameter|deadurl=
ignored (|url-status=
suggested) (help) - Costard, F.; Dupeyrat, L.; Gautier, E.; Carey-Gailhardis, E. (2003). "Fluvial thermal erosion investigations along a rapidly eroding river bank: application to the Lena River (central Siberia)". Earth Surface Processes and Landforms. 28: 1349. doi:10.1002/esp.592.
- Jones, B.M. (2008). "Modern Erosion Rates and Loss of Coastal Features and Sites, Beaufort Sea Coastline, Alaska". Arctic. 61 (4). The Arctic Institute of North America: 361–372.
{{cite journal}}
: Unknown parameter|coauthors=
ignored (|author=
suggested) (help) - IPCC. 1995. Second Assessment Synthesis of Scientific-Technical Information relevant to interpreting Article 2 of the UN Framework Convention on Climate Change. Intergovernmental Panel on Climate Change. Geneva, Switzerland. 64 pp.
- Karl, T.R. and R. W. Knight. 1998. Secular trend of precipitation amount, frequency, and intensity in the United States. Bulletin of the American Meteorological Society 79:231-242.
- Pruski, F. F. and M.A. Nearing. 2002. Runoff and soil loss responses to changes in precipitation: a computer simulation study. Journal of Soil and Water Conservation 57(1), 7-16.
- Willett, Sean D., et al., Tectonics, Climate and Landscape Evolution, Geological Society of America Special Paper 398, 2006 ISBN 978-0-8137-2398-3 PDF of Introduction
- Finnie, I., Erosion of surfaces by solid particles. Wear, 1960. 3(2): p. 87.
- ASTM G76-07
- World Bank 2001: China: Air, Land, and Water.
- Pan European Soil Erosion Assessment
Further reading
- Boardman, John (2006). Soil erosion in Europe. Chichester: Wiley. ISBN 978-0-470-85910-0.
{{cite book}}
: Unknown parameter|coauthors=
ignored (|author=
suggested) (help) - Montgomery, David R. (2007) Soil erosion and agricultural sustainability PNAS 104: 13268-13272.
- Brown, Jason (2009). Classic Erosion. Venture West: Wiley.
{{cite book}}
: Unknown parameter|coauthors=
ignored (|author=
suggested) (help)
External links
- The Soil Erosion Site
- International Erosion Control Association
- USDA National Soil Erosion Laboratory
- The Soil and Water Conservation Society
- International Soil Conservation Organization
- Bioerosion website at The College of Wooster
- Pulawy Erosion Research Center
- Southwest Watershed Research Center
River morphology | |
---|---|
Large-scale features | |
Alluvial rivers | |
Bedrock river | |
Bedforms | |
Regional processes | |
Mechanics | |