This is an old revision of this page, as edited by Muntuwandi (talk | contribs) at 16:31, 28 May 2007. The present address (URL) is a permanent link to this revision, which may differ significantly from the current revision.
Revision as of 16:31, 28 May 2007 by Muntuwandi (talk | contribs)(diff) ← Previous revision | Latest revision (diff) | Newer revision → (diff)![]() | It has been suggested that this article be merged with Race and genetics. (Discuss) |
Origins of modern humans
Any biological model for race must account for the development of racial differences during human evolution. For much of the 20th century, however, anthropologists relied on an incomplete fossil record for reconstructing human evolution. Their models seldom provided a firm basis for drawing inferences about the origin of races. Modern research in molecular biology, however, has provided evolutionary scientists with a whole new kind of data, which adds considerably to the knowledge of our past.
There has been considerable debate among anthropologists as to the origins of Homo sapiens. About a million years ago Homo erectus migrated out of Africa and into Europe and Asia. The debate hinges on whether Homo erectus evolved into Homo sapiens more or less simultaneously in Africa, Europe, and Asia, or whether Homo sapiens evolved only in Africa, and eventually supplanted Homo erectus in Europe and Asia. Each model suggests different possible scenarios for the evolution of distinct races.
Multiregional hypothesis
Main article: multiregional hypothesisAdvocates of the multiregional continuity evolution model (see Frayer et al. 1993) cite as evidence anatomical continuity in the fossil record in South Central Europe (Smith 1982), East Asia and Australia (Wolpoff 1993) (anatomical affinity is taken to suggest genetic affinity). They argue that very strong genetic similarities among all humans do not prove recent common ancestry, but rather reflect the interconnectedness of human populations around the world, resulting in relatively constant gene flow (Thorne and Wolpoff 1992). They further argue that this model is consistent with clinal patterns (Wolpoff 1993).
The most important element of this model for theories of race is that it allows a million years for the evolution of Homo sapiens around the world; this is more than enough time for the evolution of different races. Leiberman and Jackson (1995), however, have noted that this model depends on several findings relevant to race: (1) that marked morphological contrasts exist between individuals found at the center and at the perimeter of Middle Pleistocene range of the genus Homo; (2) that many features can be shown to emerge at the edge of that range before they develop at the center; and (3) that these features exhibit great tenacity through time. Regional variations in these features can thus be taken as evidence for long term differences among genus Homo individuals that prefigure different races among present-day Homo sapiens individuals.
Out of Africa
Main article: recent single-origin hypothesis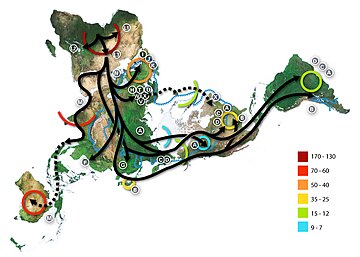
Information about the history of our species comes from two main sources: the paleoanthropological record and historical inferences based on current genetic differences observed in humans. Although both sources of information are fragmentary, they have been converging in recent years on the same general story.
Since the 1990s, it has become common to use multilocus genotypes to distinguish different human groups and to allocate individuals to groups (Bamshad et al. 2004). These data have led to an examination of the biological validity of races as evolutionary lineages and the description of races in cladistic terms. The technique of multilocus genotyping has been used to determine patterns of human demographic history. Thus, the concept of "race" afforded by these techniques is synonymous with ancestry, broadly understood.
Studies of human genetic variation imply that Africa was the ancestral source of all modern humans, and that Homo sapiens migrated out of Africa and displaced Homo erectus starting 50,000 years ago . Indigenous Australians are believed to be an early out-group that remained isolated. Most other groups, including North Africans, Eurasians , and Native Americans, were found to be a single related (monophyletic) group resulting from a later out-migration from Africa, and could reasonably be divided into West and East Eurasian subgroups.
The existing fossil evidence suggests that anatomically modern humans evolved in Africa, within the last ∼200,000 years, from a pre-existing population of humans (Klein 1999). Although it is not easy to define "anatomically modern" in a way that encompasses all living humans and excludes all archaic humans (Lieberman et al. 2002), the generally agreed-upon physical characteristics of anatomical modernity include a high rounded skull, facial retraction, and a light and gracile, as opposed to heavy and robust, skeleton (Lahr 1996). Early fossils with these characteristics have been found in eastern Africa and have been dated to ∼160,000–200,000 years ago (White et al. 2003; McDougall et al. 2005). At that time, the population of anatomically modern humans appears to have been small and localized (Harpending et al. 1998). Much larger populations of archaic humans lived elsewhere in the Old World, including the Neandertals in Europe and an earlier species of humans, Homo erectus, in Asia (Swisher et al. 1994).
Fossils of the earliest anatomically modern humans found outside Africa are from two sites in the Middle East and date to a period of relative global warmth, ∼100,000 years ago, though this region was reinhabited by Neandertals in later millennia as the climate in the northern hemisphere again cooled (Lahr and Foley 1998). Groups of anatomically modern humans appear to have moved outside Africa permanently sometime >60,000 years ago. One of the earliest modern skeletons found outside Africa is Mungo Man, from Australia, and has been dated to ∼42,000 years ago (Bowler et al. 2003), although studies of environmental changes in Australia argue for the presence of modern humans in Australia >55,000 years ago (Miller et al. 1999). To date, the earliest anatomically modern skeleton discovered from Europe comes from the Carpathian Mountains of Romania and is dated to 34,000–36,000 years ago (Trinkaus et al. 2003).
Existing data on human genetic variation support and extend conclusions based on the fossil evidence. African populations exhibit greater genetic diversity than do populations in the rest of the world, implying that humans appeared first in Africa and later colonized Eurasia and the Americas (Tishkoff and Williams 2002; Yu et al. 2002; Tishkoff and Verrelli 2003). The genetic variation seen outside Africa is generally a subset of the variation within Africa, a pattern that would be produced if the migrants from Africa were limited in number and carried just part of African genetic variability with them (Cavalli-Sforza and Feldman 2003). Patterns of genetic variation suggest an earlier population expansion in Africa followed by a subsequent expansion in non-African populations, and the dates calculated for the expansions generally coincide with the archaeological record (Jorde et al. 1998).
Aspects of the relationship between anatomically modern and archaic humans remain contentious. Studies of mtDNA (Ingman et al. 2000), the Y chromosome (Underhill et al. 2000), portions of the X chromosome (Kaessmann et al. 1999), and many (though not all) autosomal regions (Harpending and Rogers 2000) support the "Out of Africa" account of human history, in which anatomically modern humans appeared first in eastern Africa and then migrated throughout Africa and into the rest of the world, with little or no interbreeding between modern humans and the archaic populations they gradually replaced (Tishkoff et al. 2000; Stringer 2002). However, several groups of researchers cite fossil and genetic evidence to argue for a more complex account. They contend that humans bearing modern traits emerged several times from Africa, over an extended period, and mixed with archaic humans in various parts of the world (Hawks et al. 2000; Eswaran 2002; Templeton 2002; Ziętkiewicz et al. 2003). As a result, they say, autosomal DNA from archaic human populations living outside Africa persists in modern populations, and modern populations in various parts of the world still bear some physical resemblance to the archaic populations that inhabited those regions (Wolpoff et al. 2001).
However, distinguishing possible contributions to the gene pool of modern humans from archaic humans outside Africa is difficult, especially since many autosomal loci coalesce at times preceding the separation of archaic human populations (Pääbo 2003). In addition, studies of mtDNA from archaic and modern humans and extant Y chromosomes suggest that any surviving genetic contributions of archaic humans outside Africa must be small, if they exist at all (Krings et al. 1997; Nordborg 1998; Takahata et al. 2001; Serre et al. 2004). The observation that most genes studied to date coalesce in African populations points toward the importance of Africa as the source of most modern genetic variation, perhaps with some subdivision in the ancestral African population (Satta and Takahata 2002). Sequence data for hundreds of loci from widely distributed worldwide populations eventually may clarify the population processes associated with the appearance of anatomically modern humans (Wall 2000), as well as the amount of gene flow among modern humans since then.
Cladistics
A phylogenetic tree like the one shown above is usually derived from DNA or protein sequences from populations. Often mitochondrial DNA or Y chromosome sequences are used to study ancient human demographics. These single-locus sources of DNA do not recombine and are almost always inherited from a single parent, with only one known exception in mtDNA (Schwartz and Vissing 2002). Individuals from the various continental groups tend to be more similar to one another than to people from other continents. The tree is rooted in the common ancestor of chimpanzees and humans, which is believed to have originated in Africa. Horizontal distance corresponds to two things:
- Genetic distance. Given below the diagram, the genetic difference between humans and chimps is roughly 2%, or 20 times larger than the variation among modern humans.
- Temporal remoteness of the most recent common ancestor. Rough estimates are given above the diagram, in millions of years. The mitochondrial most recent common ancestor of modern humans lived roughly 200,000 years ago, latest common ancestors of humans and chimps between four and seven million years ago.
Chimpanzees and humans belong to different genera, indicated in red. Formation of species and subspecies is also indicated, and the formation of "races" is indicated in the green rectangle to the right (note that only a very rough representation of human phylogeny is given). Note that vertical distances are not meaningful in this representation.
Distribution of variation
A thorough description of the differences in patterns of genetic variation between humans and other species awaits additional genetic studies of human populations and nonhuman species. But the data gathered to date suggest that human variation exhibits several distinctive characteristics. First, compared with many other mammalian species, humans are genetically less diverse—a counterintuitive finding, given our large population and worldwide distribution (Li and Sadler 1991; Kaessmann et al. 2001). For example, the chimpanzee subspecies living just in central and western Africa have higher levels of diversity than do humans (Ebersberger et al. 2002; Yu et al. 2003; Fischer et al. 2004).
Two random humans are expected to differ at approximately 1 in 1000 nucleotides, whereas two random chimpanzees differ at 1 in 500 nucleotide pairs. However, with a genome of approximate 3 billion nucleotides, on average two humans differ at approximately 3 million nucleotides. Most of these single nucleotide polymorphisms (SNPs) are neutral, but some are functional and influence the phenotypic differences between humans. It is estimated that about 10 million SNPs exist in human populations, where the rarer SNP allele has a frequency of at least 1% (see International HapMap Project).
The distribution of variants within and among human populations also differs from that of many other species. The details of this distribution are impossible to describe succinctly because of the difficulty of defining a "population," the clinal nature of variation, and heterogeneity across the genome (Long and Kittles 2003). In general, however, 5%–15% of genetic variation occurs between large groups living on different continents, with the remaining majority of the variation occurring within such groups (Lewontin 1972; Jorde et al. 2000a; Hinds et al. 2005). This distribution of genetic variation differs from the pattern seen in many other mammalian species, for which existing data suggest greater differentiation between groups (Templeton 1998; Kittles and Weiss 2003).
Our history as a species also has left genetic signals in regional populations. For example, in addition to having higher levels of genetic diversity, populations in Africa tend to have lower amounts of linkage disequilibrium than do populations outside Africa, partly because of the larger size of human populations in Africa over the course of human history and partly because the number of modern humans who left Africa to colonize the rest of the world appears to have been relatively low (Gabriel et al. 2002). In contrast, populations that have undergone dramatic size reductions or rapid expansions in the past and populations formed by the mixture of previously separate ancestral groups can have unusually high levels of linkage disequilibrium (Nordborg and Tavare 2002).
In the field of population genetics, it is believed that the distribution of neutral polymorphisms among contemporary humans reflects human demographic history. It is believed that humans passed through a population bottleneck before a rapid expansion coinciding with migrations out of Africa leading to an African-Eurasian divergence around 100,000 years ago (ca. 5,000 generations), followed by a European-Asian divergence about 40,000 years ago (ca. 2,000 generations). Richard G. Klein, Nicholas Wade and Spencer Wells, among others, have postulated that modern humans did not leave Africa and successfully colonize the rest of the world until as recently as 50,000 years B.P., pushing back the dates for subsequent population splits as well.
The rapid expansion of a previously small population has two important effects on the distribution of genetic variation. First, the so-called founder effect occurs when founder populations bring only a subset of the genetic variation from their ancestral population. Second, as founders become more geographically separated, the probability that two individuals from different founder populations will mate becomes smaller. The effect of this assortative mating is to reduce gene flow between geographical groups, and to increase the genetic distance between groups. The expansion of humans from Africa affected the distribution of genetic variation in two other ways. First, smaller (founder) populations experience greater genetic drift because of increased fluctuations in neutral polymorphisms. Second, new polymorphisms that arose in one group were less likely to be transmitted to other groups as gene flow was restricted.
Many other geographic, climatic, and historical factors have contributed to the patterns of human genetic variation seen in the world today. For example, population processes associated with colonization, periods of geographic isolation, socially reinforced endogamy, and natural selection all have affected allele frequencies in certain populations (Jorde et al. 2000b; Bamshad and Wooding 2003). In general, however, the recency of our common ancestry and continual gene flow among human groups have limited genetic differentiation in our species.
Substructure in the human population
New data on human genetic variation has reignited the debate surrounding race. Most of the controversy surrounds the question of how to interpret these new data, and whether conclusions based on existing data are sound. A large majority of researchers endorse the view that continental groups do not constitute different subspecies. However, other researchers still debate whether evolutionary lineages should rightly be called "races". These questions are particularly pressing for biomedicine, where self-described race is often used as an indicator of ancestry (see race in biomedicine below).
Although the genetic differences among human groups are relatively small, these differences in certain genes such as duffy, ABCC11, SLC24A5, called ancestry-informative markers (AIMs) nevertheless can be used to reliably situate many individuals within broad, geographically based groupings or self-identified race. For example, computer analyses of hundreds of polymorphic loci sampled in globally distributed populations have revealed the existence of genetic clustering that roughly is associated with groups that historically have occupied large continental and subcontinental regions (Rosenberg et al. 2002; Bamshad et al. 2003).
Some commentators have argued that these patterns of variation provide a biological justification for the use of traditional racial categories. They argue that the continental clusterings correspond roughly with the division of human beings into sub-Saharan Africans; Europeans, Western Asians, Southern Asians and Northern Africans + Eastern Asians, Southeast Asians, Polynesians and Native Americans; and other inhabitants of Oceania (Melanesians, Micronesians & Australian Aborigines) (Risch et al. 2002). Other observers disagree, saying that the same data undercut traditional notions of racial groups (King and Motulsky 2002; Calafell 2003; Tishkoff and Kidd 2004). They point out, for example, that major populations considered races or subgroups within races do not necessarily form their own clusters. Thus, samples taken from India and Pakistan affiliate with Europeans or eastern Asians rather than separating into a distinct cluster.
Furthermore, because human genetic variation is clinal, many individuals affiliate with two or more continental groups. Thus, the genetically based "biogeographical ancestry" assigned to any given person generally will be broadly distributed and will be accompanied by sizable uncertainties (Pfaff et al. 2004).
In many parts of the world, groups have mixed in such a way that many individuals have relatively recent ancestors from widely separated regions. Although genetic analyses of large numbers of loci can produce estimates of the percentage of a person's ancestors coming from various continental populations (Shriver et al. 2003; Bamshad et al. 2004), these estimates may assume a false distinctiveness of the parental populations, since human groups have exchanged mates from local to continental scales throughout history (Cavalli-Sforza et al. 1994; Hoerder 2002). Even with large numbers of markers, information for estimating admixture proportions of individuals or groups is limited, and estimates typically will have wide CIs (Pfaff et al. 2004).
Physical variation in humans
Further information: ]
The distribution of many physical traits resembles the distribution of genetic variation within and between human populations (American Association of Physical Anthropologists 1996; Keita and Kittles 1997). For example, ∼90% of the variation in human head shapes occurs within every human group, and ∼10% separates groups, with a greater variability of head shape among individuals with recent African ancestors (Relethford 2002).
A prominent exception to the common distribution of physical characteristics within and among groups is skin color. Approximately 10% of the variance in skin color occurs within groups, and ~90% occurs between groups (Relethford 2002). This distribution of skin color and its geographic patterning—with people whose ancestors lived predominantly near the equator having darker skin than those with ancestors who lived predominantly in higher latitudes—indicate that this attribute has been under strong selective pressure. Darker skin appears to be strongly selected for in equatorial regions to prevent sunburn, skin cancer, the photolysis of folate, and damage to sweat glands (Sturm et al. 2001; Rees 2003). A leading hypothesis for the selection of lighter skin in higher latitudes is that it enables the body to form greater amounts of vitamin D, which helps prevent rickets (Jablonski 2004). Evidence for this includes the finding that a substantial portion of the differences of skin color between Europeans and Africans resides in a single gene, SLC24A5 the threonine-111 allele of which was found in 98.7 to 100% among several European samples, while the alanine-111 form was found in 93 to 100% of samples of Africans, East Asians and Indigenous Americans (Lamason et al. 2005). However, the vitamin D hypothesis is not universally accepted (Aoki 2002), and lighter skin in high latitudes may correspond simply to an absence of selection for dark skin (Harding et al. 2000). Melanin which serves as the pigment, is located in the epidermis of the skin, and is based on hereditary gene expression.
Because skin color has been under strong selective pressure, similar skin colors can result from convergent adaptation rather than from genetic relatedness. Sub-Saharan Africans, tribal populations from southern India, and Indigenous Australians have similar skin pigmentation, but genetically they are no more similar than are other widely separated groups. Furthermore, in some parts of the world in which people from different regions have mixed extensively, the connection between skin color and ancestry has been substantially weakened (Parra et al. 2004). In Brazil, for example, skin color is not closely associated with the percentage of recent African ancestors a person has, as estimated from an analysis of genetic variants differing in frequency among continent groups (Parra et al. 2003).
Considerable speculation has surrounded the possible adaptive value of other physical features characteristic of groups, such as the constellation of facial features observed in many eastern and northeastern Asians (Guthrie 1996). However, any given physical characteristic generally is found in multiple groups (Lahr 1996), and demonstrating that environmental selective pressures shaped specific physical features will be difficult, since such features may have resulted from sexual selection for individuals with certain appearances or from genetic drift (Roseman 2004).
References
- Altmüller J, Palmer LJ, Fischer G, Scherb H, Wjst M (2001) Genomewide scans of complex human diseases: true linkage is hard to find. Am J Hum Genet 69:936–950
- Aoki K (2002) Sexual selection as a cause of human skin colour variation: Darwin's hypothesis revisited. Ann Hum Biol 29:589–608
- Bamshad, Michael; Wooding, Stephen; Salisbury, Benjamin A.; Stephens, J. Claiborne (2004). Deconstructing The Relationship Between Genetics And Race. Nature Reviews Genetics 5, 598–609. reprint-zip
- Bamshad M, Wooding SP (2003) Signature of natural selection in the human genome. Nat Rev Genet 4:99–111
- Bamshad MJ, Wooding S, Watkins WS, Ostler CT, Batzer MA, Jorde LB (2003) Human population genetic structure and inference of group membership. Am J Hum Genet 72:578–589
- Cann, Rebecca, M. Stoneking, A. Wilson 1987 "Mitochondrial DNA and Human Evolution" in Nature 325(January) 31-36.
- Cardon LR, Abecasis GR (2003) Using haplotype blocks to map human complex trait loci. Trends Genet 19:135–140
- Cavalli-Sforza LL, Feldman MW (2003) The application of molecular genetic approaches to the study of human evolution. Nat Genet Suppl 33:266–275
- Collins FS (2004) What we do and don't know about "race," "ethnicity," genetics and health at the dawn of the genome era. Nat Genet 36:S13–S15
- Collins FS, Green ED, Guttmacher AE, Guyer MS, for the US National Human Genome Research Institute (2003) A vision for the future of genomics research. Nature 422:835–847
- Ebersberger I, Metzler D, Schwarz C, Pääbo S (2002) Genomewide comparison of DNA sequences between humans and chimpanzees. Am J Hum Genet 70:1490–1497
- Edwards, AW (2003). Human genetic diversity: Lewontin's fallacy Bioessays 25, 798–801.
- Foster MW, Sharp RR (2004) Beyond race: towards a whole-genome perspective on human populations and genetic variation. Nat Rev Genet 5:790–796
- Foster MW, Sharp RR, Freeman WL, Chino M, Bernsten D, Carter TH (1999) The role of community review in evaluating the risks of human genetic variation research. Am J Hum Genet 64:1719–1727
- Gabriel SB, Schaffner SF, Nguyen H, Moore JM, Roy J, Blumenstiel B, Higgins J, DeFelice M, Lochner A, Faggart M, Liu-Cordero SN, Rotimi C, Adeyemo A, Cooper R, Ward R, Lander ES, Daly MJ, Altshuler D (2002) The structure of haplotype blocks in the human genome. Science 296:2225–2229
- Harding RM, Healy E, Ray AJ, Ellis NS, Flanagan N, Todd C, Dixon C, Sajantila A, Jackson IJ, Birch-Machin MA, Rees JL (2000) Evidence for variable selective pressures at MC1R. Am J Hum Genet 66:1351–1361
- Ingman M, Kaessmann H, Pääbo S, Gyllensten U (2000) Mitochondrial genome variation and the origin of modern humans. Nature 408:708–713
- International HapMap Consortium (2003) The International HapMap Project. Nature 426:789–796
- ——— (2004) Integrating ethics and science in the International HapMap Project. Nat Rev Genet 5:467–475
- International Human Genome Sequencing Consortium (2001) Initial sequencing and analysis of the human genome. Nature 409:860–921
- Jorde LB, Bamshad M, Rogers AR (1998) Using mitochondrial and nuclear DNA markers to reconstruct human evolution. BioEssays 20:126–136
- Jorde LB, Watkins WS, Bamshad MJ, Dixon ME, Ricker CE, Seielstad MT, Batzer MA (2000a) The distribution of human genetic diversity: a comparison of mitochondrial, autosomal, and Y-chromosome data. Am J Hum Genet 66:979–988
- Jorde LB, Watkins WS, Kere J, Nyman D, Eriksson AW (2000b) Gene mapping in isolated populations: new roles for old friends? Hum Hered 50:57–65
- Jorde, Lynn B.; Wooding, Stephen P. (2004). Genetic variation, classification and race. Nature Genetics 36, S28–S33.
- Kaessmann H, Heissig F, von Haeseler A, Pääbo S (1999) DNA sequence variation in a non-coding region of low recombination on the human X chromosome. Nat Genet 22:78–81
- Kaessmann H, Wiebe V, Weiss G, Pääbo S (2001) Great ape DNA sequences reveal a reduced diversity and an expansion in humans. Nat Genet 27:155–156
- Lewontin RC (1972) The apportionment of human diversity. Evol Biol 6:381–398
- Mountain JL, Risch N (2004) Assessing genetic contributions to phenotypic differences among "racial" and "ethnic" groups. Nat Genet Suppl 36:S48–S53
- Pääbo S (2003) The mosaic that is our genome. Nature 421:409–412
- Sankar P, Cho MK (2002) Toward a new vocabulary of human genetic variation. Science 298:1337–1338
- Sankar P, Cho MK, Condit DM, Hunt LM, Koenig B, Marshall P, Lee SS, Spicer P (2004) Genetic research and health disparities. JAMA 291:2985–2989
- Serre D, Pääbo S (2004) Evidence for gradients of human genetic diversity within and among continents. Genome Res 14:1679–1685 PDF
- Weiss KM (1998) Coming to terms with human variation. Annu Rev Anthropol 27:273–300
- Weiss KM, Terwilliger JD (2000) How many diseases does it take to map a gene with SNPs? Nat Genet 26:151–157
- Yu N, Jensen-Seaman MI, Chemnick L, Kidd JR, Deinard AS, Ryder O, Kidd KK, Li WH (2003) Low nucleotide diversity in chimpanzees and bonobos. Genetics 164:1511–1518
- Ziętkiewicz E, Yotova V, Gehl D, Wambach T, Arrieta I, Batzer M, Cole DEC, Hechtman P, Kaplan F, Modiano D, Moisan J-P, Michalski R, Labuda D (2003) Haplotypes in the dystrophin DNA segment point to a mosaic origin of modern human diversity. Am J Hum Genet 73:994–1015