This is an old revision of this page, as edited by Maury Markowitz (talk | contribs) at 20:08, 26 October 2006 (rewrite). The present address (URL) is a permanent link to this revision, which may differ significantly from the current revision.
Revision as of 20:08, 26 October 2006 by Maury Markowitz (talk | contribs) (rewrite)(diff) ← Previous revision | Latest revision (diff) | Newer revision → (diff)Plasma acceleration is a technique for accelerating charged particles, such as electrons, positrons and ions, using an electric field associated with an electron plasma wave. The wave is often created by the passage of a very brief laser pulse though the plasma, which leads to the laser-wakefield accelerator for one popular type of device. The technique appears to offer a way to build high performance particle accelerators of much smaller size than conventional devices. Current experimental devices offer acceleration potentials several orders of magnitude better than current particle accelerators, for instance, one experimental device at the Lawrence Berkeley National Laboratory creates a field of 1 GeV over about 3.3 cm, whereas the SLAC device requires 64 m to reach the same energy.
Basic concept
A plasma consists of fluid of positive and negative charged particles, generally created by heating a dilute gas. Under normal conditions the plasma will be macroscopically neutral, an equal mix of electrons and nucleons in equilibrium. However, if an external electric field is applied, the plasma will separate, with the particles being attracted to the external field. A particle injected into such a plasma would be accelerated by the charge separation, but since the magnitude of this separation is generally similar to that of the external field, nothing is gained in comparison to a system that simply applies the field directly to the particle, which is the case in existing accelerator designs.
What makes the system potentially useful is the possibility of introducing waves of very high charge separation that travel through the plasma. Such a wave can be created by applying a high-power laser or electron pulse into a properly prepared plasma. As the pulse travels though the plasma, the electric field of the light separates the electrons and nucleons in the same way that an external field would. However, as the electrons are much lighter than the nucleons, they move much further during this short period, leading to a charge separation as in the case of an external field. As the laser pulse leaves the vicinity, the electrons relax into their original positions, pulled by the now-remaining positive charge of the ions that did not move. For a brief period when the reach the center, the electron density is higher than it would be normally. Although the particles are not moving very rapidly, macroscopically it appears that a powerful area of negative electrical force is traveling through the plasma very close to the speed of light.
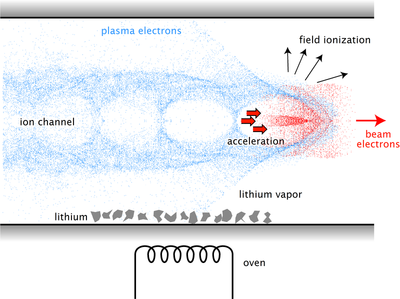
It is this "wakefield" that is used for particle acceleration. A particle injected into the plasma near the high-density area will experience an acceleration toward (or away) from it, an acceleration that continues as the wakefield travels through the column, eventually reaching the speed of the wakefield. Even higher energies can be reached by injecting the particle to travel across the face of the wakefield, much like a surfer can travel at speeds much higher than the wave they surf on by traveling across it. Accelerators designed to take advantage of this technique are generally referred to as surfatrons.
The advantage of plasma acceleration is that its acceleration field can be much stronger than that of conventional radio-frequency (RF) accelerators. In RF accelerators, the field has an upper limit determined by the threshold for dielectric breakdown of the acceleration tube. This limits the amount of acceleration over any given area, requiring very long accelerators to reach high energies. In contrast, the maximum field in a plasma is defined by mechanical qualities and turbulance, but is generally several orders of magnitude stronger than with RF accelerators. It is hoped that a compact particle accelerator can be created based on plasma acceleration techniques or accelerators for much higher energy can be built, if long accelerators are realizable with an accelerating field of 10 GeV/m.
Plasma acceleration is categorized into several types according to how the electron plasma wave is formed: plasma wakefield acceleration (PWFA), laser wakefield acceleration (LWFA), laser beat-wave acceleration (LBWA), and self-modulated laser wakefield acceleration (SMLWFA). In PWFA, an electron plasma wave is formed by an electron bunch. In LWFA, a laser pulse is introduced to form an electron plasma wave. In LBWA, an electron plasma wave arises based on different frequency generation of two laser pulses. And in SMLWFA, the formation of an electron plasma wave is achieved by a laser pulse modulated by stimulated Raman forward scattering instability.
The concept of plasma acceleration was first proposed by Toshiki Tajima and John Dawson in a theoretical article published in 1979. The first experimental demonstration of wakefield acceleration, which was performed with PWFA, was reported by a research group at Argonne National Laboratory in 1988.
Formula
According to the acceleration gradient for a linear plasma wave is:
In this equation, electric field, is the speed of light in vacuum, is the mass of the electron, is the plasma density, and is the permittivity of free space.
is theExternal links
- http://home.fnal.gov/~carrigan/Accelerators/Accelerator_master.htm
- http://pbpl.physics.ucla.edu/Research/Experiments/Advanced_Accelerators/Plasma_Acceleration/PBWA/
- http://icfa-usa.jlab.org/archive/newsletter/icfa_bd_nl_12/node26.html