This is an old revision of this page, as edited by Bonpetin (talk | contribs) at 01:54, 4 April 2023 (←Removed redirect to Climate change). The present address (URL) is a permanent link to this revision, which may differ significantly from the current revision.
Revision as of 01:54, 4 April 2023 by Bonpetin (talk | contribs) (←Removed redirect to Climate change)(diff) ← Previous revision | Latest revision (diff) | Newer revision → (diff) Current rise in Earth's average temperature and its effects "Global warming" redirects here. For other uses, see Climate change (disambiguation) and Global warming (disambiguation). This redirect is about contemporary climate change. For historical climate trends, see Climate variability and change.
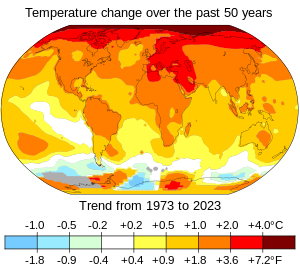

In common usage, climate change describes global warming—the ongoing increase in global average temperature—and its effects on Earth's climate system. Climate change in a broader sense also includes previous long-term changes to Earth's climate. The current rise in global average temperature is more rapid than previous changes, and is primarily caused by humans burning fossil fuels. Fossil fuel use, deforestation, and some agricultural and industrial practices increase greenhouse gases, notably carbon dioxide and methane. Greenhouse gases absorb some of the heat that the Earth radiates after it warms from sunlight. Larger amounts of these gases trap more heat in Earth's lower atmosphere, causing global warming.
Due to climate change, deserts are expanding, while heat waves and wildfires are becoming more common. Increased warming in the Arctic has contributed to melting permafrost, glacial retreat and sea ice loss. Higher temperatures are also causing more intense storms, droughts, and other weather extremes. Rapid environmental change in mountains, coral reefs, and the Arctic is forcing many species to relocate or become extinct. Even if efforts to minimise future warming are successful, some effects will continue for centuries. These include ocean heating, ocean acidification and sea level rise.
Climate change threatens people with increased flooding, extreme heat, increased food and water scarcity, more disease, and economic loss. Human migration and conflict can also be a result. The World Health Organization (WHO) calls climate change the greatest threat to global health in the 21st century. Societies and ecosystems will experience more severe risks in the future without action to limit warming. Adapting to climate change through efforts like flood control measures or drought-resistant crops reduces climate change risks, although this may not be possible with increasing warming. Poorer countries are responsible for a small share of global emissions, yet they have the least ability to adapt and are most vulnerable to climate change.
Many climate change impacts are already felt at the current 1.2 °C (2.2 °F) level of warming. Additional warming will increase these impacts and can trigger tipping points, such as the melting of the Greenland ice sheet. Under the 2015 Paris Agreement, nations collectively agreed to keep warming "well under 2 °C". However, with pledges made under the Agreement, global warming would still reach about 2.7 °C (4.9 °F) by the end of the century. Limiting warming to 1.5 °C will require halving emissions by 2030 and achieving net-zero emissions by 2050.



Reducing emissions requires generating electricity from low-carbon sources rather than burning fossil fuels. This change includes phasing out coal and natural gas fired power plants, vastly increasing use of wind, solar, and other types of renewable energy, and reducing energy use. Electricity generated from non-carbon-emitting sources will need to replace fossil fuels for powering transportation, heating buildings, and operating industrial facilities. Carbon can also be removed from the atmosphere, for instance by increasing forest cover and by farming with methods that capture carbon in soil.
Terminology
Before the 1980s, when it was unclear whether the warming effect of increased greenhouse gases were stronger than the cooling effect of airborne particulates in air pollution, scientists used the term inadvertent climate modification to refer to human impacts on the climate.
In the 1980s, the terms global warming and climate change became more common. Though the two terms are sometimes used interchangeably, scientifically, global warming refers only to increased surface warming, while climate change describes the totality of changes to Earth's climate system. Global warming—used as early as 1975—became the more popular term after NASA climate scientist James Hansen used it in his 1988 testimony in the U.S. Senate. Since the 2000s, climate change has increased in usage. Climate change can also refer more broadly to both human-caused changes or natural changes throughout Earth's history.
Various scientists, politicians and media now use the terms climate crisis or climate emergency to talk about climate change, and global heating instead of global warming.
Observed temperature rise
Main articles: Temperature record of the last 2,000 years and Instrumental temperature record
Multiple independent instrumental datasets show that the climate system is warming. The 2011–2020 decade warmed to an average 1.09 °C compared to the pre-industrial baseline (1850–1900). Surface temperatures are rising by about 0.2 °C per decade, with 2020 reaching a temperature of 1.2 °C above the pre-industrial era. Since 1950, the number of cold days and nights has decreased, and the number of warm days and nights has increased.
There was little net warming between the 18th century and the mid-19th century. Climate information for that period comes from climate proxies, such as trees and ice cores. Thermometer records began to provide global coverage around 1850. Historical patterns of warming and cooling, like the Medieval Climate Anomaly and the Little Ice Age, did not occur at the same time across different regions. Temperatures may have reached as high as those of the late-20th century in a limited set of regions. There have been prehistorical episodes of global warming, such as the Paleocene–Eocene Thermal Maximum. However, the modern observed rise in temperature and CO2 concentrations has been so rapid that even abrupt geophysical events in Earth's history do not approach current rates.
Evidence of warming from air temperature measurements are reinforced with a wide range of other observations. For example, changes to the natural water cycle have been predicted and observed, such as an increase in the frequency and intensity of heavy precipitation, melting of snow and land ice, and increased atmospheric humidity. Flora and fauna are also behaving in a manner consistent with warming; for instance, plants are flowering earlier in spring. Another key indicator is the cooling of the upper atmosphere, which demonstrates that greenhouse gases are trapping heat near the Earth's surface and preventing it from radiating into space.
Regions of the world warm at differing rates. The pattern is independent of where greenhouse gases are emitted, because the gases persist long enough to diffuse across the planet. Since the pre-industrial period, the average surface temperature over land regions has increased almost twice as fast as the global-average surface temperature. This is because of the larger heat capacity of oceans, and because oceans lose more heat by evaporation. The thermal energy in the global climate system has grown with only brief pauses since at least 1970, and over 90% of this extra energy has been stored in the ocean. The rest has heated the atmosphere, melted ice, and warmed the continents.
The Northern Hemisphere and the North Pole have warmed much faster than the South Pole and Southern Hemisphere. The Northern Hemisphere not only has much more land, but also more seasonal snow cover and sea ice. As these surfaces flip from reflecting a lot of light to being dark after the ice has melted, they start absorbing more heat. Local black carbon deposits on snow and ice also contribute to Arctic warming. Arctic temperatures are increasing at over twice the rate of the rest of the world. Melting of glaciers and ice sheets in the Arctic disrupts ocean circulation, including a weakened Gulf Stream, further changing the climate.
Attribution of recent temperature rise
Main article: Attribution of recent climate change
The climate system experiences various cycles on its own which can last for years (such as the El Niño–Southern Oscillation (ENSO)), decades or even centuries. Other changes are caused by an imbalance of energy that is "external" to the climate system, but not always external to the Earth. Examples of external forcings include changes in the concentrations of greenhouse gases, solar luminosity, volcanic eruptions, and variations in the Earth's orbit around the Sun.
To determine the human contribution to climate change, known internal climate variability and natural external forcings need to be ruled out. A key approach is to determine unique "fingerprints" for all potential causes, then compare these fingerprints with observed patterns of climate change. For example, solar forcing can be ruled out as a major cause. Its fingerprint would be warming in the entire atmosphere. Yet, only the lower atmosphere has warmed, consistent with greenhouse gas forcing. Attribution of recent climate change shows that the main driver is elevated greenhouse gases, with aerosols having a dampening effect.
Greenhouse gases
Main articles: Greenhouse gas, Greenhouse gas emissions, Greenhouse effect, and Carbon dioxide in Earth's atmosphere
Greenhouse gases are transparent to sunlight, and thus allow it to pass through the atmosphere to heat the Earth's surface. The Earth radiates it as heat, and greenhouse gases absorb a portion of it. This absorption slows the rate at which heat escapes into space, trapping heat near the Earth's surface and warming it over time. Before the Industrial Revolution, naturally-occurring amounts of greenhouse gases caused the air near the surface to be about 33 °C warmer than it would have been in their absence. While water vapour (~50%) and clouds (~25%) are the biggest contributors to the greenhouse effect, they increase as a function of temperature and are therefore feedbacks. On the other hand, concentrations of gases such as CO2 (~20%), tropospheric ozone, CFCs and nitrous oxide are not temperature-dependent, and are therefore external forcings.
Human activity since the Industrial Revolution, mainly extracting and burning fossil fuels (coal, oil, and natural gas), has increased the amount of greenhouse gases in the atmosphere, resulting in a radiative imbalance. In 2019, the concentrations of CO2 and methane had increased by about 48% and 160%, respectively, since 1750. These CO2 levels are higher than they have been at any time during the last 2 million years. Concentrations of methane are far higher than they were over the last 800,000 years.

Global anthropogenic greenhouse gas emissions in 2019 were equivalent to 59 billion tonnes of CO2. Of these emissions, 75% was CO2, 18% was methane, 4% was nitrous oxide, and 2% was fluorinated gases. CO2 emissions primarily come from burning fossil fuels to provide energy for transport, manufacturing, heating, and electricity. Additional CO2 emissions come from deforestation and industrial processes, which include the CO2 released by the chemical reactions for making cement, steel, aluminum, and fertiliser. Methane emissions come from livestock, manure, rice cultivation, landfills, wastewater, and coal mining, as well as oil and gas extraction. Nitrous oxide emissions largely come from the microbial decomposition of fertiliser.
Despite the contribution of deforestation to greenhouse gas emissions, the Earth's land surface, particularly its forests, remain a significant carbon sink for CO2. Land-surface sink processes, such as carbon fixation in the soil and photosynthesis, remove about 29% of annual global CO2 emissions. The ocean also serves as a significant carbon sink via a two-step process. First, CO2 dissolves in the surface water. Afterwards, the ocean's overturning circulation distributes it deep into the ocean's interior, where it accumulates over time as part of the carbon cycle. Over the last two decades, the world's oceans have absorbed 20 to 30% of emitted CO2.
Aerosols and clouds
Air pollution, in the form of aerosols, affects the climate on a large scale. Aerosols scatter and absorb solar radiation. From 1961 to 1990, a gradual reduction in the amount of sunlight reaching the Earth's surface was observed. This phenomenon is popularly known as global dimming, and is attributed to aerosols produced by dust, pollution and combustion of biofuels and fossil fuels. Globally, aerosols have been declining since 1990 due to pollution controls, meaning that they no longer mask greenhouse gas warming as much.
Aerosols also have indirect effects on the Earth's radiation budget. Sulfate aerosols act as cloud condensation nuclei and lead to clouds that have more and smaller cloud droplets. These clouds reflect solar radiation more efficiently than clouds with fewer and larger droplets. They also reduce the growth of raindrops, which makes clouds more reflective to incoming sunlight. Indirect effects of aerosols are the largest uncertainty in radiative forcing.
While aerosols typically limit global warming by reflecting sunlight, black carbon in soot that falls on snow or ice can contribute to global warming. Not only does this increase the absorption of sunlight, it also increases melting and sea-level rise. Limiting new black carbon deposits in the Arctic could reduce global warming by 0.2 °C by 2050.
Land surface changes

Humans change the Earth's surface mainly to create more agricultural land. Today, agriculture takes up 34% of Earth's land area, while 26% is forests, and 30% is uninhabitable (glaciers, deserts, etc.). The amount of forested land continues to decrease, which is the main land use change that causes global warming. Deforestation releases CO2 contained in trees when they are destroyed, plus it prevents those trees from absorbing more CO2 in the future. The main causes of deforestation are: permanent land-use change from forest to agricultural land producing products such as beef and palm oil (27%), logging to produce forestry/forest products (26%), short term shifting cultivation (24%), and wildfires (23%).
The type of vegetation in a region affects the local temperature. It impacts how much of the sunlight gets reflected back into space (albedo), and how much heat is lost by evaporation. For instance, the change from a dark forest to grassland makes the surface lighter, causing it to reflect more sunlight. Deforestation can also affect temperatures by modifying the release of chemical compounds that influence clouds, and by changing wind patterns. In tropic and temperate areas the net effect is to produce significant warming, while at latitudes closer to the poles a gain of albedo (as forest is replaced by snow cover) leads to a cooling effect. Globally, these effects are estimated to have led to a slight cooling, dominated by an increase in surface albedo. According to FAO, forest degradation aggravates the impacts of climate change as it reduces the carbon sequestration abilities of forests. Indeed, among their many benefits, forests also have the potential to reduce the impact of high temperatures.
Solar and volcanic activity
Further information: Solar activity and climateAs the Sun is the Earth's primary energy source, changes in incoming sunlight directly affect the climate system. Solar irradiance has been measured directly by satellites, and indirect measurements are available from the early 1600s onwards. There has been no upward trend in the amount of the Sun's energy reaching the Earth.
Explosive volcanic eruptions represent the largest natural forcing over the industrial era. When the eruption is sufficiently strong (with sulfur dioxide reaching the stratosphere), sunlight can be partially blocked for a couple of years. The temperature signal lasts about twice as long. In the industrial era, volcanic activity has had negligible impacts on global temperature trends. Present-day volcanic CO2 emissions are equivalent to less than 1% of current anthropogenic CO2 emissions.
Physical climate models are unable to reproduce the rapid warming observed in recent decades when taking into account only variations in solar output and volcanic activity. Further evidence for greenhouse gases causing global warming comes from measurements that show a warming of the lower atmosphere (the troposphere), coupled with a cooling of the upper atmosphere (the stratosphere). If solar variations were responsible for the observed warming, the troposphere and stratosphere would both warm.
Climate change feedback
Main articles: Climate change feedback and Climate sensitivity
The response of the climate system to an initial forcing is modified by feedbacks: increased by "self-reinforcing" or "positive" feedbacks and reduced by "balancing" or "negative" feedbacks. The main reinforcing feedbacks are the water-vapour feedback, the ice–albedo feedback, and the net effect of clouds. The primary balancing mechanism is radiative cooling, as Earth's surface gives off more heat to space in response to rising temperature. In addition to temperature feedbacks, there are feedbacks in the carbon cycle, such as the fertilizing effect of CO2 on plant growth. Uncertainty over feedbacks is the major reason why different climate models project different magnitudes of warming for a given amount of emissions.
As air warms, it can hold more moisture. Water vapour, as a potent greenhouse gas, holds heat in the atmosphere. If cloud cover increases, more sunlight will be reflected back into space, cooling the planet. If clouds become higher and thinner, they act as an insulator, reflecting heat from below back downwards and warming the planet. The effect of clouds is the largest source of feedback uncertainty.
Another major feedback is the reduction of snow cover and sea ice in the Arctic, which reduces the reflectivity of the Earth's surface. More of the Sun's energy is now absorbed in these regions, contributing to amplification of Arctic temperature changes. Arctic amplification is also melting permafrost, which releases methane and CO2 into the atmosphere. Climate change can also cause methane releases from wetlands, marine systems, and freshwater systems. Overall, climate feedbacks are expected to become increasingly positive.
Around half of human-caused CO2 emissions have been absorbed by land plants and by the oceans. On land, elevated CO2 and an extended growing season have stimulated plant growth. Climate change increases droughts and heat waves that inhibit plant growth, which makes it uncertain whether this carbon sink will continue to grow in the future. Soils contain large quantities of carbon and may release some when they heat up. As more CO2 and heat are absorbed by the ocean, it acidifies, its circulation changes and phytoplankton takes up less carbon, decreasing the rate at which the ocean absorbs atmospheric carbon. Overall, at higher CO2 concentrations the Earth will absorb a reduced fraction of our emissions.
Modelling
Further information: Carbon budget, Climate model, and Climate change scenario
A climate model is a representation of the physical, chemical, and biological processes that affect the climate system. Models also include natural processes like changes in the Earth's orbit, historical changes in the Sun's activity, and volcanic forcing. Models are used to estimate the degree of warming future emissions will cause when accounting for the strength of climate feedbacks, or reproduce and predict the circulation of the oceans, the annual cycle of the seasons, and the flows of carbon between the land surface and the atmosphere.
The physical realism of models is tested by examining their ability to simulate contemporary or past climates. Past models have underestimated the rate of Arctic shrinkage and underestimated the rate of precipitation increase. Sea level rise since 1990 was underestimated in older models, but more recent models agree well with observations. The 2017 United States-published National Climate Assessment notes that "climate models may still be underestimating or missing relevant feedback processes".
A subset of climate models add societal factors to a simple physical climate model. These models simulate how population, economic growth, and energy use affect – and interact with – the physical climate. With this information, these models can produce scenarios of future greenhouse gas emissions. This is then used as input for physical climate models and carbon cycle models to predict how atmospheric concentrations of greenhouse gases might change in the future. Depending on the socioeconomic scenario and the mitigation scenario, models produce atmospheric CO2 concentrations that range widely between 380 and 1400 ppm.
The IPCC Sixth Assessment Report projects that global warming is very likely to reach 1.0 °C to 1.8 °C by the late 21st century under the very low GHG emissions scenario. In an intermediate scenario global warming would reach 2.1 °C to 3.5 °C, and 3.3 °C to 5.7 °C under the very high GHG emissions scenario. These projections are based on climate models in combination with observations.
The remaining carbon budget is determined by modelling the carbon cycle and the climate sensitivity to greenhouse gases. According to the IPCC, global warming can be kept below 1.5 °C with a two-thirds chance if emissions after 2018 do not exceed 420 or 570 gigatonnes of CO2. This corresponds to 10 to 13 years of current emissions. There are high uncertainties about the budget. For instance, it may be 100 gigatonnes of CO2 smaller due to methane release from permafrost and wetlands. However, it is clear that fossil fuel resources are too abundant for shortages to be relied on to limit carbon emissions in the 21st century.
Impacts
Main article: Effects of climate change
Environmental effects
Further information: Effects of climate change on oceans and Effects of climate change on the water cycleThe environmental effects of climate change are broad and far-reaching, affecting oceans, ice, and weather. Changes may occur gradually or rapidly. Evidence for these effects comes from studying climate change in the past, from modelling, and from modern observations. Since the 1950s, droughts and heat waves have appeared simultaneously with increasing frequency. Extremely wet or dry events within the monsoon period have increased in India and East Asia. The rainfall rate and intensity of hurricanes and typhoons is likely increasing, and the geographic range likely expanding poleward in response to climate warming. Frequency of tropical cyclones has not increased as a result of climate change.

Global sea level is rising as a consequence of glacial melt, melt of the ice sheets in Greenland and Antarctica, and thermal expansion. Between 1993 and 2020, the rise increased over time, averaging 3.3 ± 0.3 mm per year. Over the 21st century, the IPCC projects that in a very high emissions scenario the sea level could rise by 61–110 cm. Increased ocean warmth is undermining and threatening to unplug Antarctic glacier outlets, risking a large melt of the ice sheet and the possibility of a 2-meter sea level rise by 2100 under high emissions.
Climate change has led to decades of shrinking and thinning of the Arctic sea ice. While ice-free summers are expected to be rare at 1.5 °C degrees of warming, they are set to occur once every three to ten years at a warming level of 2 °C. Higher atmospheric CO2 concentrations have led to changes in ocean chemistry. An increase in dissolved CO2 is causing oceans to acidify. In addition, oxygen levels are decreasing as oxygen is less soluble in warmer water. Dead zones in the ocean, regions with very little oxygen, are expanding too.
Tipping points and long-term impacts
Greater degrees of global warming increase the risk of passing through ‘tipping points’—thresholds beyond which certain impacts can no longer be avoided even if temperatures are reduced. An example is the collapse of West Antarctic and Greenland ice sheets, where a temperature rise of 1.5 to 2 °C may commit the ice sheets to melt, although the time scale of melt is uncertain and depends on future warming. Some large-scale changes could occur over a short time period, such as a shutdown of certain ocean currents like the Atlantic Meridional Overturning Circulation (AMOC). Tipping points can also include irreversible damage to ecosystems like the Amazon rainforest and coral reefs.
The long-term effects of climate change on oceans include further ice melt, ocean warming, sea level rise, and ocean acidification. On the timescale of centuries to millennia, the magnitude of climate change will be determined primarily by anthropogenic CO2 emissions. This is due to CO2's long atmospheric lifetime. Oceanic CO2 uptake is slow enough that ocean acidification will continue for hundreds to thousands of years. These emissions are estimated to have prolonged the current interglacial period by at least 100,000 years. Sea level rise will continue over many centuries, with an estimated rise of 2.3 metres per degree Celsius (4.2 ft/°F) after 2000 years.
Nature and wildlife
Further information: Effects of climate change on oceans and Climate change and ecosystemsRecent warming has driven many terrestrial and freshwater species poleward and towards higher altitudes. Higher atmospheric CO2 levels and an extended growing season have resulted in global greening. However, heatwaves and drought have reduced ecosystem productivity in some regions. The future balance of these opposing effects is unclear. Climate change has contributed to the expansion of drier climate zones, such as the expansion of deserts in the subtropics. The size and speed of global warming is making abrupt changes in ecosystems more likely. Overall, it is expected that climate change will result in the extinction of many species.
The oceans have heated more slowly than the land, but plants and animals in the ocean have migrated towards the colder poles faster than species on land. Just as on land, heat waves in the ocean occur more frequently due to climate change, harming a wide range of organisms such as corals, kelp, and seabirds. Ocean acidification makes it harder for marine calcifying organisms such as mussels, barnacles and corals to produce shells and skeletons; and heatwaves have bleached coral reefs. Harmful algal blooms enhanced by climate change and eutrophication lower oxygen levels, disrupt food webs and cause great loss of marine life. Coastal ecosystems are under particular stress. Almost half of global wetlands have disappeared due to climate change and other human impacts.
|
Humans
Main article: Effects of climate change Further information: Effects of climate change on human health, Climate security, Economics of climate change, and Effects of climate change on agriculture
The effects of climate change are impacting humans everywhere in the world. Impacts can now be observed on all continents and ocean regions, with low-latitude, less developed areas facing the greatest risk. Continued warming has potentially “severe, pervasive and irreversible impacts” for people and ecosystems. The risks are unevenly distributed, but are generally greater for disadvantaged people in developing and developed countries.
Food and health
The WHO has classified climate change as the greatest threat to global health in the 21st century. Extreme weather leads to injury and loss of life, and crop failures to undernutrition. Various infectious diseases are more easily transmitted in a warmer climate, such as dengue fever and malaria. Young children are the most vulnerable to food shortages. Both children and older people are vulnerable to extreme heat. The World Health Organization (WHO) has estimated that between 2030 and 2050, climate change would cause around 250,000 additional deaths per year. They assessed deaths from heat exposure in elderly people, increases in diarrhea, malaria, dengue, coastal flooding, and childhood undernutrition. Over 500,000 more adult deaths are projected yearly by 2050 due to reductions in food availability and quality. By 2100, 50% to 75% of the global population may face climate conditions that are life-threatening due to combined effects of extreme heat and humidity.
Climate change is affecting food security. It has caused reduction in global yields of maize, wheat, and soybeans between 1981 and 2010. Future warming could further reduce global yields of major crops. Crop production will probably be negatively affected in low-latitude countries, while effects at northern latitudes may be positive or negative. Up to an additional 183 million people worldwide, particularly those with lower incomes, are at risk of hunger as a consequence of these impacts. Climate change also impacts fish populations. Globally, less will be available to be fished. Regions dependent on glacier water, regions that are already dry, and small islands have a higher risk of water stress due to climate change.
Livelihoods
Economic damages due to climate change may be severe and there is a chance of disastrous consequences. Climate change has likely already increased global economic inequality, and this trend is projected to continue. Most of the severe impacts are expected in sub-Saharan Africa, where most of the local inhabitants are dependent upon natural and agricultural resources and South-East Asia. The World Bank estimates that climate change could drive over 120 million people into poverty by 2030.
Current inequalities based on wealth and social status have worsened due to climate change. Major difficulties in mitigating, adapting, and recovering to climate shocks are faced by marginalized people who have less control over resources. Indigenous people, who are subsistent on their land and ecosystems, will face endangerment to their wellness and lifestyles due to climate change. An expert elicitation concluded that the role of climate change in armed conflict has been small compared to factors such as socio-economic inequality and state capabilities.
Low-lying islands and coastal communities are threatened by sea level rise, which makes flooding more common. Sometimes, land is permanently lost to the sea. This could lead to statelessness for people in island nations, such as the Maldives and Tuvalu. In some regions, the rise in temperature and humidity may be too severe for humans to adapt to. With worst-case climate change, models project that almost one-third of humanity might live in extremely hot and uninhabitable climates, similar to the current climate found in the Sahara. These factors can drive environmental migration, both within and between countries. More people are expected to be displaced because of sea level rise, extreme weather and conflict from increased competition over natural resources. Climate change may also increase vulnerability, leading to "trapped populations" who are not able to move due to a lack of resources.
|
Reducing and recapturing emissions
Main article: Climate change mitigation
Climate change can be mitigated by reducing greenhouse gas emissions and enhancing sinks that absorb greenhouse gases from the atmosphere. In order to limit global warming to less than 1.5 °C global greenhouse gas emissions needs to be net-zero by 2050, or by 2070 with a 2 °C target. This requires far-reaching, systemic changes on an unprecedented scale in energy, land, cities, transport, buildings, and industry. The United Nations Environment Programme estimates that countries need to triple their pledges under the Paris Agreement within the next decade to limit global warming to 2 °C. An even greater level of reduction is required to meet the 1.5 °C goal. With pledges made under the Agreement as of October 2021, global warming would still have a 66% chance of reaching about 2.7 °C (range: 2.2–3.2 °C) by the end of the century. Globally, limiting warming to 2 °C may result in higher benefits than costs.
Although there is no single pathway to limit global warming to 1.5 or 2 °C, most scenarios and strategies see a major increase in the use of renewable energy in combination with increased energy efficiency measures to generate the needed greenhouse gas reductions. To reduce pressures on ecosystems and enhance their carbon sequestration capabilities, changes would also be necessary in agriculture and forestry, such as preventing deforestation and restoring natural ecosystems by reforestation.
Other approaches to mitigating climate change have a higher level of risk. Scenarios that limit global warming to 1.5 °C typically project the large-scale use of carbon dioxide removal methods over the 21st century. There are concerns, though, about over-reliance on these technologies, and environmental impacts. Solar radiation modification (SRM) is also a possible supplement to deep reductions in emissions. However, SRM would raise significant ethical and legal issues, and the risks are poorly understood.
Clean energy
Main articles: Sustainable energy and Sustainable transport

Renewable energy is key to limiting climate change. Fossil fuels accounted for 80% of the world's energy in 2018. The remaining share was split between nuclear power and renewables (including hydropower, bioenergy, wind and solar power and geothermal energy). That mix is projected to change significantly over the next 30 years. Solar panels and onshore wind are now among the cheapest forms of adding new power generation capacity in many locations. Renewables represented 75% of all new electricity generation installed in 2019, nearly all solar and wind. Other forms of clean energy, such as nuclear and hydropower, currently have a larger share of the energy supply. However, their future growth forecasts appear limited in comparison.
To achieve carbon neutrality by 2050, renewable energy would become the dominant form of electricity generation, rising to 85% or more by 2050 in some scenarios. Investment in coal would be eliminated and coal use nearly phased out by 2050.
Electricity generated from renewable sources would also need to become the main energy source for heating and transport. Transport can switch away from internal combustion engine vehicles and towards electric vehicles, public transit, and active transport (cycling and walking). For shipping and flying, low-carbon fuels would reduce emissions. Heating could be increasingly decarbonised with technologies like heat pumps.
There are obstacles to the continued rapid growth of clean energy, including renewables. For wind and solar, there are environmental and land use concerns for new projects. Wind and solar also produce energy intermittently and with seasonal variability. Traditionally, hydro dams with reservoirs and conventional power plants have been used when variable energy production is low. Going forward, battery storage can be expanded, energy demand and supply can be matched, and long-distance transmission can smooth variability of renewable outputs. Bioenergy is often not carbon-neutral and may have negative consequences for food security. The growth of nuclear power is constrained by controversy around nuclear waste, nuclear weapon proliferation, and accidents. Hydropower growth is limited by the fact that the best sites have been developed, and new projects are confronting increased social and environmental concerns.
Low-carbon energy improves human health by minimising climate change. It also has the near-term benefit of reducing air pollution deaths, which were estimated at 7 million annually in 2016. Meeting the Paris Agreement goals that limit warming to a 2 °C increase could save about a million of those lives per year by 2050, whereas limiting global warming to 1.5 °C could save millions and simultaneously increase energy security and reduce poverty. Improving air quality also has economic benefits which may be larger than mitigation costs.
Energy conservation
Main articles: Efficient energy use and Energy conservationReducing energy demand is another major aspect of reducing emissions. If less energy is needed, there is more flexibility for clean energy development. It also makes it easier to manage the electricity grid, and minimises carbon-intensive infrastructure development. Major increases in energy efficiency investment will be required to achieve climate goals, comparable to the level of investment in renewable energy. Several COVID-19 related changes in energy use patterns, energy efficiency investments, and funding have made forecasts for this decade more difficult and uncertain.
Strategies to reduce energy demand vary by sector. In transport, passengers and freight can switch to more efficient travel modes, such as buses and trains, or use electric vehicles. Industrial strategies to reduce energy demand include improving heating systems and motors, designing less energy-intensive products, and increasing product lifetimes. In the building sector the focus is on better design of new buildings, and higher levels of energy efficiency in retrofitting. The use of technologies like heat pumps can also increase building energy efficiency.
Agriculture and industry
See also: Sustainable agriculture and Green industrial policy
Agriculture and forestry face a triple challenge of limiting greenhouse gas emissions, preventing the further conversion of forests to agricultural land, and meeting increases in world food demand. A set of actions could reduce agriculture and forestry-based emissions by two thirds from 2010 levels. These include reducing growth in demand for food and other agricultural products, increasing land productivity, protecting and restoring forests, and reducing greenhouse gas emissions from agricultural production.
On the demand side, a key component of reducing emissions is shifting people towards plant-based diets. Eliminating the production of livestock for meat and dairy would eliminate about 3/4ths of all emissions from agriculture and other land use. Livestock also occupy 37% of ice-free land area on Earth and consume feed from the 12% of land area used for crops, driving deforestation and land degradation.
Steel and cement production are responsible for about 13% of industrial CO2 emissions. In these industries, carbon-intensive materials such as coke and lime play an integral role in the production, so that reducing CO2 emissions requires research into alternative chemistries.
Carbon sequestration
Main articles: Carbon dioxide removal and Carbon sequestration
Natural carbon sinks can be enhanced to sequester significantly larger amounts of CO2 beyond naturally occurring levels. Reforestation and tree planting on non-forest lands are among the most mature sequestration techniques, although the latter raises food security concerns. Farmers can promote sequestration of carbon in soils through practices such as use of winter cover crops, reducing the intensity and frequency of tillage, and using compost and manure as soil amendments. In one of its recent publications, FAO maintains that forest and landscape restoration yields many benefits for the climate, including greenhouse gas emissions sequestration and reduction. Restoration/recreation of coastal wetlands and seagrass meadows increases the uptake of carbon into organic matter (blue carbon). When carbon is sequestered in soils and in organic matter such as trees, there is a risk of the carbon being re-released into the atmosphere later through changes in land use, fire, or other changes in ecosystems.
Where energy production or CO2-intensive heavy industries continue to produce waste CO2, the gas can be captured and stored instead of released to the atmosphere. Although its current use is limited in scale and expensive, carbon capture and storage (CCS) may be able to play a significant role in limiting CO2 emissions by mid-century. This technique, in combination with bioenergy (BECCS) can result in net negative emissions: CO2 is drawn from the atmosphere. It remains highly uncertain whether carbon dioxide removal techniques will be able to play a large role in limiting warming to 1.5 °C. Policy decisions that rely on carbon dioxide removal increase the risk of global warming rising beyond international goals.
Adaptation
Main article: Climate change adaptation


Adaptation is "the process of adjustment to current or expected changes in climate and its effects". Without additional mitigation, adaptation cannot avert the risk of "severe, widespread and irreversible" impacts. More severe climate change requires more transformative adaptation, which can be prohibitively expensive. The capacity and potential for humans to adapt is unevenly distributed across different regions and populations, and developing countries generally have less. The first two decades of the 21st century saw an increase in adaptive capacity in most low- and middle-income countries with improved access to basic sanitation and electricity, but progress is slow. Many countries have implemented adaptation policies. However, there is a considerable gap between necessary and available finance.
Adaptation to sea level rise consists of avoiding at-risk areas, learning to live with increased flooding and protection. If that fails, managed retreat may be needed. There are economic barriers for tackling dangerous heat impact. Avoiding strenuous work or having air conditioning is not possible for everybody. In agriculture, adaptation options include a switch to more sustainable diets, diversification, erosion control and genetic improvements for increased tolerance to a changing climate. Insurance allows for risk-sharing, but is often difficult to get for people on lower incomes. Education, migration and early warning systems can reduce climate vulnerability. Planting mangroves or encouraging other coastal vegetation can buffer storms.
Ecosystems adapt to climate change, a process that can be supported by human intervention. By increasing connectivity between ecosystems, species can migrate to more favourable climate conditions. Species can also be introduced to areas acquiring a favorable climate. Protection and restoration of natural and semi-natural areas helps build resilience, making it easier for ecosystems to adapt. Many of the actions that promote adaptation in ecosystems, also help humans adapt via ecosystem-based adaptation. For instance, restoration of natural fire regimes makes catastrophic fires less likely, and reduces human exposure. Giving rivers more space allows for more water storage in the natural system, reducing flood risk. Restored forest acts as a carbon sink, but planting trees in unsuitable regions can exacerbate climate impacts.
There are synergies but also trade-offs between adaptation and mitigation. Adaptation often offer short-term benefits, whereas mitigation has longer-term benefits. Two examples for trade-offs include: Increased use of air conditioning allows people to better cope with heat, but increases energy demand. Compact urban development may lead to reduced emissions from transport and construction. At the same time, this kind of urban development may increase the urban heat island effect, leading to higher temperatures and increased exposure. An example for synergy is increased food productivity which has large benefits for both adaptation and mitigation.
Policies and politics
Main article: Politics of climate change
High | Medium | Low | Very Low |
Countries that are most vulnerable to climate change have typically been responsible for a small share of global emissions. This raises questions about justice and fairness. Climate change is strongly linked to sustainable development. Limiting global warming makes it easier to achieve sustainable development goals, such as eradicating poverty and reducing inequalities. The connection is recognised in Sustainable Development Goal 13 which is to "take urgent action to combat climate change and its impacts". The goals on food, clean water and ecosystem protection have synergies with climate mitigation.
The geopolitics of climate change is complex. It has often been framed as a free-rider problem, in which all countries benefit from mitigation done by other countries, but individual countries would lose from switching to a low-carbon economy themselves. This framing has been challenged. For instance, the benefits of a coal phase-out to public health and local environments exceed the costs in almost all regions. Furthermore, net importers of fossil fuels win economically from switching to clean energy, causing net exporters to face stranded assets: fossil fuels they cannot sell.
Policy options
A wide range of policies, regulations, and laws are being used to reduce emissions. As of 2019, carbon pricing covers about 20% of global greenhouse gas emissions. Carbon can be priced with carbon taxes and emissions trading systems. Direct global fossil fuel subsidies reached $319 billion in 2017, and $5.2 trillion when indirect costs such as air pollution are priced in. Ending these can cause a 28% reduction in global carbon emissions and a 46% reduction in air pollution deaths. Money saved on fossil subsidies could be used to support the transition to clean energy instead. More direct methods to reduce greenhouse gases include vehicle efficiency standards, renewable fuel standards, and air pollution regulations on heavy industry. Several countries require utilities to increase the share of renewables in power production.
Policy designed through the lens of climate justice tries to address human rights issues and social inequality. For instance, wealthy nations responsible for the largest share of emissions would have to pay poorer countries to adapt. As the use of fossil fuels is reduced, jobs in the sector are being lost. To achieve a just transition, these people would need to be retrained for other jobs. Communities with many fossil fuel workers would need additional investments.
International climate agreements
Further information: United Nations Framework Convention on Climate Change

Nearly all countries in the world are parties to the 1994 United Nations Framework Convention on Climate Change (UNFCCC). The goal of the UNFCCC is to prevent dangerous human interference with the climate system. As stated in the convention, this requires that greenhouse gas concentrations are stabilised in the atmosphere at a level where ecosystems can adapt naturally to climate change, food production is not threatened, and economic development can be sustained. The UNFCCC does not itself restrict emissions but rather provides a framework for protocols that do. Global emissions have risen since the UNFCCC was signed. Its yearly conferences are the stage of global negotiations.
The 1997 Kyoto Protocol extended the UNFCCC and included legally binding commitments for most developed countries to limit their emissions. During the negotiations, the G77 (representing developing countries) pushed for a mandate requiring developed countries to " the lead" in reducing their emissions, since developed countries contributed most to the accumulation of greenhouse gases in the atmosphere. Per-capita emissions were also still relatively low in developing countries and developing countries would need to emit more to meet their development needs.
The 2009 Copenhagen Accord has been widely portrayed as disappointing because of its low goals, and was rejected by poorer nations including the G77. Associated parties aimed to limit the global temperature rise to below 2 °C. The Accord set the goal of sending $100 billion per year to developing countries for mitigation and adaptation by 2020, and proposed the founding of the Green Climate Fund. As of 2020, the fund has failed to reach its expected target, and risks a shrinkage in its funding.
In 2015 all UN countries negotiated the Paris Agreement, which aims to keep global warming well below 2.0 °C and contains an aspirational goal of keeping warming under 1.5 °C. The agreement replaced the Kyoto Protocol. Unlike Kyoto, no binding emission targets were set in the Paris Agreement. Instead, a set of procedures was made binding. Countries have to regularly set ever more ambitious goals and reevaluate these goals every five years. The Paris Agreement restated that developing countries must be financially supported. As of October 2021, 194 states and the European Union have signed the treaty and 191 states and the EU have ratified or acceded to the agreement.
The 1987 Montreal Protocol, an international agreement to stop emitting ozone-depleting gases, may have been more effective at curbing greenhouse gas emissions than the Kyoto Protocol specifically designed to do so. The 2016 Kigali Amendment to the Montreal Protocol aims to reduce the emissions of hydrofluorocarbons, a group of powerful greenhouse gases which served as a replacement for banned ozone-depleting gases. This made the Montreal Protocol a stronger agreement against climate change.
National responses
In 2019, the United Kingdom parliament became the first national government to declare a climate emergency. Other countries and jurisdictions followed suit. That same year, the European Parliament declared a "climate and environmental emergency". The European Commission presented its European Green Deal with the goal of making the EU carbon-neutral by 2050. Major countries in Asia have made similar pledges: South Korea and Japan have committed to become carbon-neutral by 2050, and China by 2060. In 2021, the European Commission released its “Fit for 55” legislation package, which contains guidelines for the car industry; all new cars on the European market must be zero-emission vehicles from 2035. While India has strong incentives for renewables, it also plans a significant expansion of coal in the country. Vietnam is among very few coal-dependent fast developing countries that pledged to phase out unabated coal power by the 2040s or as soon as possible there after.
As of 2021, based on information from 48 national climate plans, which represent 40% of the parties to the Paris Agreement, estimated total greenhouse gas emissions will be 0.5% lower compared to 2010 levels, below the 45% or 25% reduction goals to limit global warming to 1.5 °C or 2 °C, respectively.
Society
Denial and misinformation
Further information: Global warming controversy, Fossil fuels lobby, Climate change denial, and Global warming conspiracy theory
Public debate about climate change has been strongly affected by climate change denial and misinformation, which originated in the United States and has since spread to other countries, particularly Canada and Australia. The actors behind climate change denial form a well-funded and relatively coordinated coalition of fossil fuel companies, industry groups, conservative think tanks, and contrarian scientists. Like the tobacco industry, the main strategy of these groups has been to manufacture doubt about scientific data and results. Many who deny, dismiss, or hold unwarranted doubt about the scientific consensus on anthropogenic climate change are labelled as "climate change skeptics", which several scientists have noted is a misnomer.
There are different variants of climate denial: some deny that warming takes place at all, some acknowledge warming but attribute it to natural influences, and some minimise the negative impacts of climate change. Manufacturing uncertainty about the science later developed into a manufactured controversy: creating the belief that there is significant uncertainty about climate change within the scientific community in order to delay policy changes. Strategies to promote these ideas include criticism of scientific institutions, and questioning the motives of individual scientists. An echo chamber of climate-denying blogs and media has further fomented misunderstanding of climate change.
Public awareness and opinion
Further information: Climate communication, Media coverage of climate change, and Public opinion on climate change
Climate change came to international public attention in the late 1980s. Due to media coverage in the early 1990s, people often confused climate change with other environmental issues like ozone depletion. In popular culture, the climate fiction movie The Day After Tomorrow (2004) and the Al Gore documentary An Inconvenient Truth (2006) focused on climate change.
Significant regional, gender, age and political differences exist in both public concern for, and understanding of, climate change. More highly educated people, and in some countries, women and younger people, were more likely to see climate change as a serious threat. Partisan gaps also exist in many countries, and countries with high CO2 emissions tend to be less concerned. Views on causes of climate change vary widely between countries. Concern has increased over time, to the point where in 2021 a majority of citizens in many countries express a high level of worry about climate change, or view it as a global emergency. Higher levels of worry are associated with stronger public support for policies that address climate change.
Climate movement
Main articles: Climate movement and Climate change litigationClimate protests demand that political leaders take action to prevent climate change. They can take the form of public demonstrations, fossil fuel divestment, lawsuits and other activities. Prominent demonstrations include the School Strike for Climate. In this initiative, young people across the globe have been protesting since 2018 by skipping school on Fridays, inspired by Swedish teenager Greta Thunberg. Mass civil disobedience actions by groups like Extinction Rebellion have protested by disrupting roads and public transport. Litigation is increasingly used as a tool to strengthen climate action from public institutions and companies. Activists also initiate lawsuits which target governments and demand that they take ambitious action or enforce existing laws on climate change. Lawsuits against fossil-fuel companies generally seek compensation for loss and damage.
History
For broader coverage of this topic, see History of climate change science.Early discoveries

Scientists in the 19th century such as Alexander von Humboldt began to foresee the effects of climate change. In the 1820s, Joseph Fourier proposed the greenhouse effect to explain why Earth's temperature was higher than the sun's energy alone could explain. Earth's atmosphere is transparent to sunlight, so sunlight reaches the surface where it is converted to heat. However, the atmosphere is not transparent to heat radiating from the surface, and captures some of that heat, which in turn warms the planet.
In 1856 Eunice Newton Foote demonstrated that the warming effect of the sun is greater for air with water vapour than for dry air, and that the effect is even greater with carbon dioxide (CO2). She concluded that "An atmosphere of that gas would give to our earth a high temperature..."
Starting in 1859, John Tyndall established that nitrogen and oxygen—together totaling 99% of dry air—are transparent to radiated heat. However, water vapour and gases such as methane and carbon dioxide absorb radiated heat and re-radiate that heat into the atmosphere. Tyndall proposed that changes in the concentrations of these gases may have caused climatic changes in the past, including ice ages.
Svante Arrhenius noted that water vapour in air continuously varied, but the CO2 concentration in air was influenced by long-term geological processes. Warming from increased CO2 levels would increase the amount of water vapour, amplifying warming in a positive feedback loop. In 1896, he published the first climate model of its kind, projecting that halving CO2 levels could have produced a drop in temperature initiating an ice age. Arrhenius calculated the temperature increase expected from doubling CO2 to be around 5–6 °C. Other scientists were initially skeptical and believed that the greenhouse effect was saturated so that adding more CO2 would make no difference, and that the climate would be self-regulating. Beginning in 1938, Guy Stewart Callendar published evidence that climate was warming and CO2 levels were rising, but his calculations met the same objections.
Development of a scientific consensus
See also: Scientific consensus on climate changeIn the 1950s, Gilbert Plass created a detailed computer model that included different atmospheric layers and the infrared spectrum. This model predicted that increasing CO2 levels would cause warming. Around the same time, Hans Suess found evidence that CO2 levels had been rising, and Roger Revelle showed that the oceans would not absorb the increase. The two scientists subsequently helped Charles Keeling to begin a record of continued increase, which has been termed the "Keeling Curve". Scientists alerted the public, and the dangers were highlighted at James Hansen's 1988 Congressional testimony. The Intergovernmental Panel on Climate Change (IPCC), set up in 1988 to provide formal advice to the world's governments, spurred interdisciplinary research. As part of the IPCC reports, scientists assess the scientific discussion that takes place in peer-reviewed journal articles.
There is a near-complete scientific consensus that the climate is warming and that this is caused by human activities. As of 2019, agreement in recent literature reached over 99%. No scientific body of national or international standing disagrees with this view. Consensus has further developed that some form of action should be taken to protect people against the impacts of climate change. National science academies have called on world leaders to cut global emissions. The 2021 IPCC Assessment Report stated that it is "unequivocal" that climate change is caused by humans.
See also
- Anthropocene – proposed new geological time interval in which humans are having significant geological impact
- List of climate scientists
References
- IPCC AR6 WG1 2021, SPM-7
- IPCC SR15 Ch1 2018, p. 54: Since 1970 the global average temperature has been rising at a rate of 1.7°C per century, compared to a long-term decline over the past 7,000 years at a baseline rate of 0.01°C per century (NOAA, 2016; Marcott et al., 2013). These global-level rates of human-driven change far exceed the rates of change driven by geophysical or biosphere forces that have altered the Earth System trajectory in the past (e.g., Summerhayes, 2015; Foster et al., 2017); even abrupt geophysical events do not approach current rates of human-driven change.
- ^ Lynas, Mark; Houlton, Benjamin Z.; Perry, Simon (19 October 2021). "Greater than 99% consensus on human caused climate change in the peer-reviewed scientific literature". Environmental Research Letters. 16 (11): 114005. Bibcode:2021ERL....16k4005L. doi:10.1088/1748-9326/ac2966. S2CID 239032360.
- ^ Our World in Data, 18 September 2020
- IPCC SRCCL 2019, p. 7: Since the pre-industrial period, the land surface air temperature has risen nearly twice as much as the global average temperature (high confidence). Climate change... contributed to desertification and land degradation in many regions (high confidence).; IPCC SRCCL 2019, p. 45: Climate change is playing an increasing role in determining wildfire regimes alongside human activity (medium confidence), with future climate variability expected to enhance the risk and severity of wildfires in many biomes such as tropical rainforests (high confidence).
- IPCC SROCC 2019, p. 16: Over the last decades, global warming has led to widespread shrinking of the cryosphere, with mass loss from ice sheets and glaciers (very high confidence), reductions in snow cover (high confidence) and Arctic sea ice extent and thickness (very high confidence), and increased permafrost temperature (very high confidence).
- IPCC AR6 WG1 Ch11 2021, p. 1517
- EPA (19 January 2017). "Climate Impacts on Ecosystems". Archived from the original on 27 January 2018. Retrieved 5 February 2019.
Mountain and arctic ecosystems and species are particularly sensitive to climate change... As ocean temperatures warm and the acidity of the ocean increases, bleaching and coral die-offs are likely to become more frequent.
- IPCC SR15 Ch1 2018, p. 64: Sustained net zero anthropogenic emissions of CO2 and declining net anthropogenic non-CO2 radiative forcing over a multi-decade period would halt anthropogenic global warming over that period, although it would not halt sea level rise or many other aspects of climate system adjustment.
- ^ Cattaneo et al. 2019; IPCC AR6 WG2 2022, p. 15; 53
- IPCC AR5 SYR 2014, pp. 13–16; WHO, Nov 2015: "Climate change is the greatest threat to global health in the 21st century. Health professionals have a duty of care to current and future generations. You are on the front line in protecting people from climate impacts – from more heat-waves and other extreme weather events; from outbreaks of infectious diseases such as malaria, dengue and cholera; from the effects of malnutrition; as well as treating people who are affected by cancer, respiratory, cardiovascular and other non-communicable diseases caused by environmental pollution."
- IPCC AR6 WG2 2022, p. 19
- IPCC AR6 WG2 2022, pp. 21–26, 2504
- IPCC AR6 WG1 Technical Summary 2021, p. 71
- ^ United Nations Environment Programme 2021, p. 36: "A continuation of the effort implied by the latest unconditional NDCs and announced pledges is at present estimated to result in warming of about 2.7 °C (range: 2.2–3.2 °C) with a 66 per cent chance."
- IPCC SR15 Ch2 2018, pp. 95–96: In model pathways with no or limited overshoot of 1.5 °C, global net anthropogenic CO2 emissions decline by about 45% from 2010 levels by 2030 (40–60% interquartile range), reaching net zero around 2050 (2045–2055 interquartile range); IPCC SR15 2018, p. 17, SPM C.3:All pathways that limit global warming to 1.5 °C with limited or no overshoot project the use of carbon dioxide removal (CDR) on the order of 100–1000 GtCO2 over the 21st century. CDR would be used to compensate for residual emissions and, in most cases, achieve net negative emissions to return global warming to 1.5 °C following a peak (high confidence). CDR deployment of several hundreds of GtCO2 is subject to multiple feasibility and sustainability constraints (high confidence).; Rogelj et al. 2015; Hilaire et al. 2019
- Ivanova, Irina (2 June 2022). "California is rationing water amid its worst drought in 1,200 years". CBS News.
- United Nations Environment Programme 2019, p. xxiii, Table ES.3; Teske, ed. 2019, p. xxvii, Fig.5.
- United Nations Environment Programme 2019, Table ES.3 & p. 49; NREL 2017, pp. vi, 12
- ^ IPCC SRCCL Summary for Policymakers 2019, p. 18
- ^ NASA, 5 December 2008.
- NASA, 7 July 2020; Shaftel 2016: " 'Climate change' and 'global warming' are often used interchangeably but have distinct meanings. ... Global warming refers to the upward temperature trend across the entire Earth since the early 20th century ... Climate change refers to a broad range of global phenomena ... include the increased temperature trends described by global warming."; Associated Press, 22 September 2015: "The terms global warming and climate change can be used interchangeably. Climate change is more accurate scientifically to describe the various effects of greenhouse gases on the world because it includes extreme weather, storms and changes in rainfall patterns, ocean acidification and sea level.".
- Broeker, Wallace S. (8 August 1975). "Climatic Change: Are We on the Brink of a Pronounced Global Warming?". Science. 189 (4201): 460–463. Bibcode:1975Sci...189..460B. doi:10.1126/science.189.4201.460. JSTOR 1740491. PMID 17781884. S2CID 16702835.
- ^ Weart "The Public and Climate Change: The Summer of 1988", "News reporters gave only a little attention ...".
- Joo et al. 2015.
- IPCC AR5 SYR Glossary 2014, p. 120: "Climate change refers to a change in the state of the climate that can be identified (e.g., by using statistical tests) by changes in the mean and/or the variability of its properties and that persists for an extended period, typically decades or longer. Climate change may be due to natural internal processes or external forcings such as modulations of the solar cycles, volcanic eruptions and persistent anthropogenic changes in the composition of the atmosphere or in land use."
- Hodder & Martin 2009; BBC Science Focus Magazine, 3 February 2020
- Neukom et al. 2019b.
- "Global Annual Mean Surface Air Temperature Change". NASA. Retrieved 23 February 2020.
- EPA 2016: The U.S. Global Change Research Program, the National Academy of Sciences, and the Intergovernmental Panel on Climate Change (IPCC) have each independently concluded that warming of the climate system in recent decades is "unequivocal". This conclusion is not drawn from any one source of data but is based on multiple lines of evidence, including three worldwide temperature datasets showing nearly identical warming trends as well as numerous other independent indicators of global warming (e.g. rising sea levels, shrinking Arctic sea ice).
- IPCC AR6 WG1 Summary for Policymakers 2021, p. SPM-5
- IPCC SR15 Ch1 2018, p. 81.
- WMO 2021, p. 6.
- IPCC AR5 WG1 Ch2 2013, p. 162.
- IPCC SR15 Ch1 2018, p. 57: This report adopts the 51-year reference period, 1850–1900 inclusive, assessed as an approximation of pre-industrial levels in AR5 ... Temperatures rose by 0.0 °C–0.2 °C from 1720–1800 to 1850–1900; Hawkins et al. 2017, p. 1844
- IPCC AR5 WG1 Summary for Policymakers 2013, pp. 4–5: "Global-scale observations from the instrumental era began in the mid-19th century for temperature and other variables ... the period 1880 to 2012 ... multiple independently produced datasets exist."
- IPCC AR5 WG1 Ch5 2013, p. 386; Neukom et al. 2019a
- IPCC AR5 WG1 Ch5 2013, pp. 389, 399–400: "The PETM was marked by ... global warming of 4 °C to 7 °C ... Deglacial global warming occurred in two main steps from 17.5 to 14.5 ka and 13.0 to 10.0 ka."
- IPCC SR15 Ch1 2018, p. 54.
- Kennedy et al. 2010, p. S26. Figure 2.5.
- Loeb et al. 2021.
- Kennedy et al. 2010, pp. S26, S59–S60; USGCRP Chapter 1 2017, p. 35.
- IPCC AR4 WG2 Ch1 2007, p. 99, Sec. 1.3.5.1
- "Global Warming". NASA JPL. 3 June 2010. Retrieved 11 September 2020.
Satellite measurements show warming in the troposphere but cooling in the stratosphere. This vertical pattern is consistent with global warming due to increasing greenhouse gases but inconsistent with warming from natural causes.
- IPCC SRCCL Summary for Policymakers 2019, p. 7
- Sutton, Dong & Gregory 2007.
- "Climate Change: Ocean Heat Content". Noaa Climate.gov. NOAA. 2018. Archived from the original on 12 February 2019. Retrieved 20 February 2019.
- IPCC AR5 WG1 Ch3 2013, p. 257: "Ocean warming dominates the global energy change inventory. Warming of the ocean accounts for about 93% of the increase in the Earth's energy inventory between 1971 and 2010 (high confidence), with warming of the upper (0 to 700 m) ocean accounting for about 64% of the total.
- von Schuckman, K.; Cheng, L.; Palmer, M. D.; Hansen, J.; et al. (7 September 2020). "Heat stored in the Earth system: where does the energy go?". Earth System Science Data. 12 (3): 2013–2041. Bibcode:2020ESSD...12.2013V. doi:10.5194/essd-12-2013-2020.
- NOAA, 10 July 2011.
- United States Environmental Protection Agency 2016, p. 5: "Black carbon that is deposited on snow and ice darkens those surfaces and decreases their reflectivity (albedo). This is known as the snow/ice albedo effect. This effect results in the increased absorption of radiation that accelerates melting."
- IPCC AR5 WG1 Ch12 2013, p. 1062; IPCC SROCC Ch3 2019, p. 212.
- NASA, 12 September 2018.
- Delworth & Zeng 2012, p. 5; Franzke et al. 2020
- National Research Council 2012, p. 9
- IPCC AR5 WG1 Ch10 2013, p. 916.
- Knutson 2017, p. 443; IPCC AR5 WG1 Ch10 2013, pp. 875–876
- ^ USGCRP 2009, p. 20.
- IPCC AR5 WG1 Summary for Policymakers 2013, pp. 13–14
- Lüthi, Dieter; Le Floch, Martine; Bereiter, Bernhard; Blunier, Thomas; Barnola, Jean-Marc; Siegenthaler, Urs; Raynaud, Dominique; Jouzel, Jean; Fischer, Hubertus; Kawamura, Kenji; Stocker, Thomas F. (May 2005). "High-resolution carbon dioxide concentration record 650,000–800,000 years before present". Nature. 453 (7193): 379–382. doi:10.1038/nature06949. ISSN 0028-0836. PMID 18480821. S2CID 1382081.
- Fischer, Hubertus; Wahlen, Martin; Smith, Jesse; Mastroianni, Derek; Deck, Bruce (12 March 1999). "Ice Core Records of Atmospheric CO 2 Around the Last Three Glacial Terminations". Science. 283 (5408): 1712–1714. Bibcode:1999Sci...283.1712F. doi:10.1126/science.283.5408.1712. ISSN 0036-8075. PMID 10073931.
- Indermühle, Andreas; Monnin, Eric; Stauffer, Bernhard; Stocker, Thomas F.; Wahlen, Martin (1 March 2000). "Atmospheric CO 2 concentration from 60 to 20 kyr BP from the Taylor Dome Ice Core, Antarctica". Geophysical Research Letters. 27 (5): 735–738. Bibcode:2000GeoRL..27..735I. doi:10.1029/1999GL010960. S2CID 18942742.
- Etheridge, D.; Steele, L.; Langenfelds, R.; Francey, R.; Barnola, J.-M.; Morgan, V. (1998). "Historical CO2 Records from the Law Dome DE08, DE08-2, and DSS Ice Cores". Carbon Dioxide Information Analysis Center, Oak Ridge National Laboratory. U.S. Department of Energy. Retrieved 20 November 2022.
- Keeling, C.; Whorf, T. (2004). "Atmospheric CO2 Records from Sites in the SIO Air Sampling Network". Carbon Dioxide Information Analysis Center, Oak Ridge National Laboratory. U.S. Department of Energy. Retrieved 20 November 2022.
- NASA. "The Causes of Climate Change". Climate Change: Vital Signs of the Planet. Archived from the original on 8 May 2019. Retrieved 8 May 2019.
- IPCC AR4 WG1 Ch1 2007, FAQ1.1: "To emit 240 W m, a surface would have to have a temperature of around −19 °C. This is much colder than the conditions that actually exist at the Earth's surface (the global mean surface temperature is about 14 °C).
- ACS. "What Is the Greenhouse Effect?". Archived from the original on 26 May 2019. Retrieved 26 May 2019.
- Ozone acts as a greenhouse gas in the lowest layer of the atmosphere, the troposphere (as opposed to the stratospheric ozone layer). Wang, Shugart & Lerdau 2017
- Schmidt et al. 2010; USGCRP Climate Science Supplement 2014, p. 742
- The Guardian, 19 February 2020.
- WMO 2021, p. 8.
- IPCC AR6 WG1 Technical Summary 2021, p. TS-35.
- IPCC AR6 WG3 Summary for Policymakers 2022, Figure SPM.1.
- Olivier & Peters 2019, p. 17; Our World in Data, 18 September 2020; EPA 2020: Greenhouse gas emissions from industry primarily come from burning fossil fuels for energy, as well as greenhouse gas emissions from certain chemical reactions necessary to produce goods from raw materials; "Redox, extraction of iron and transition metals".
Hot air (oxygen) reacts with the coke (carbon) to produce carbon dioxide and heat energy to heat up the furnace. Removing impurities: The calcium carbonate in the limestone thermally decomposes to form calcium oxide. calcium carbonate → calcium oxide + carbon dioxide
; Kvande 2014: Carbon dioxide gas is formed at the anode, as the carbon anode is consumed upon reaction of carbon with the oxygen ions from the alumina (Al2O3). Formation of carbon dioxide is unavoidable as long as carbon anodes are used, and it is of great concern because CO2 is a greenhouse gas - EPA 2020; Global Methane Initiative 2020: Estimated Global Anthropogenic Methane Emissions by Source, 2020: Enteric fermentation (27%), Manure Management (3%), Coal Mining (9%), Municipal Solid Waste (11%), Oil & Gas (24%), Wastewater (7%), Rice Cultivation (7%)
- EPA 2019: Agricultural activities, such as fertilizer use, are the primary source of N2O emissions; Davidson 2009: 2.0% of manure nitrogen and 2.5% of fertilizer nitrogen was converted to nitrous oxide between 1860 and 2005; these percentage contributions explain the entire pattern of increasing nitrous oxide concentrations over this period
- IPCC SRCCL Summary for Policymakers 2019, p. 10
- IPCC SROCC Ch5 2019, p. 450.
- Haywood 2016, p. 456; McNeill 2017; Samset et al. 2018.
- IPCC AR5 WG1 Ch2 2013, p. 183.
- He et al. 2018; Storelvmo et al. 2016
- "Global 'Sunscreen' Has Likely Thinned, Report NASA Scientists". NASA. 15 March 2007.
- "Aerosol pollution has caused decades of global dimming".
- Xia, Wenwen; Wang, Yong; Chen, Siyu; Huang, Jianping; Wang, Bin; Zhang, Guang J.; Zhang, Yue; Liu, Xiaohong; Ma, Jianmin; Gong, Peng; Jiang, Yiquan; Wu, Mingxuan; Xue, Jinkai; Wei, Linyi; Zhang, Tinghan (2022). "Double Trouble of Air Pollution by Anthropogenic Dust". Environmental Science & Technology. 56 (2): 761–769. Bibcode:2022EnST...56..761X. doi:10.1021/acs.est.1c04779. hdl:10138/341962. PMID 34941248.
- "Global Dimming Dilemma". 4 June 2020.
- Wild et al. 2005; Storelvmo et al. 2016; Samset et al. 2018.
- Twomey 1977.
- Albrecht 1989.
- ^ USGCRP Chapter 2 2017, p. 78.
- Ramanathan & Carmichael 2008; RIVM 2016.
- Sand et al. 2015
- World Resources Institute, 31 March 2021
- Ritchie & Roser 2018
- The Sustainability Consortium, 13 September 2018; UN FAO 2016, p. 18.
- Curtis et al. 2018
- ^ World Resources Institute, 8 December 2019
- IPCC SRCCL Ch2 2019, p. 172: "The global biophysical cooling alone has been estimated by a larger range of climate models and is −0.10 ± 0.14 °C; it ranges from −0.57 °C to +0.06°C ... This cooling is essentially dominated by increases in surface albedo: historical land cover changes have generally led to a dominant brightening of land"
- ^ Garrett, L.; Lévite, H.; Besacier, C.; Alekseeva, N.; Duchelle, M. (2022). The key role of forest and landscape restoration in climate action. Rome: FAO. ISBN 978-92-5-137044-5.
- National Academies 2008, p. 6
- "Is the Sun causing global warming?". Climate Change: Vital Signs of the Planet. Archived from the original on 5 May 2019. Retrieved 10 May 2019.
- USGCRP Chapter 2 2017, p. 79
- Fischer & Aiuppa 2020.
- Schmidt, Shindell & Tsigaridis 2014; Fyfe et al. 2016.
- IPCC AR4 WG1 Ch9 2007, pp. 702–703; Randel et al. 2009.
- "Thermodynamics: Albedo". NSIDC. Archived from the original on 11 October 2017. Retrieved 10 October 2017.
- "The study of Earth as an integrated system". Vitals Signs of the Planet. Earth Science Communications Team at NASA's Jet Propulsion Laboratory / California Institute of Technology. 2013. Archived from the original on 26 February 2019.
- ^ USGCRP Chapter 2 2017, pp. 89–91.
- IPCC AR6 WG1 Technical Summary 2021, p. 58: The net effect of changes in clouds in response to global warming is to amplify human-induced warming, that is, the net cloud feedback is positive (high confidence)
- USGCRP Chapter 2 2017, pp. 89–90.
- IPCC AR5 WG1 2013, p. 14
- Wolff et al. 2015: "the nature and magnitude of these feedbacks are the principal cause of uncertainty in the response of Earth's climate (over multi-decadal and longer periods) to a particular emissions scenario or greenhouse gas concentration pathway."
- Williams, Ceppi & Katavouta 2020.
- IPCC AR6 WG1 Technical Summary 2021, p. 58,59: clouds remain the largest contribution to overall uncertainty in climate feedbacks
- NASA, 28 May 2013.
- Cohen et al. 2014.
- ^ Turetsky et al. 2019
- Dean et al. 2018.
- IPCC AR6 WG1 Technical Summary 2021, p. 58: Feedback processes are expected to become more positive overall (more amplifying of global surface temperature changes) on multi-decadal time scales as the spatial pattern of surface warming evolves and global surface temperature increases.
- NASA, 16 June 2011: "So far, land plants and the ocean have taken up about 55 percent of the extra carbon people have put into the atmosphere while about 45 percent has stayed in the atmosphere. Eventually, the land and oceans will take up most of the extra carbon dioxide, but as much as 20 percent may remain in the atmosphere for many thousands of years."
- IPCC SRCCL Ch2 2019, pp. 133, 144.
- Melillo et al. 2017: Our first-order estimate of a warming-induced loss of 190 Pg of soil carbon over the 21st century is equivalent to the past two decades of carbon emissions from fossil fuel burning.
- USGCRP Chapter 2 2017, pp. 93–95.
- IPCC AR6 WG1 Technical Summary 2021, p. TS-122, Box TS.5, Figure 1
- IPCC AR5 SYR Glossary 2014, p. 120.
- Carbon Brief, 15 January 2018, "What are the different types of climate models?"
- Wolff et al. 2015
- Carbon Brief, 15 January 2018, "Who does climate modelling around the world?"
- Carbon Brief, 15 January 2018, "What is a climate model?"
- IPCC AR4 WG1 Ch8 2007, FAQ 8.1.
- Stroeve et al. 2007; National Geographic, 13 August 2019
- Liepert & Previdi 2009.
- Rahmstorf et al. 2007; Mitchum et al. 2018
- USGCRP Chapter 15 2017.
- Carbon Brief, 15 January 2018, "What are the inputs and outputs for a climate model?"
- Matthews et al. 2009
- Carbon Brief, 19 April 2018; Meinshausen 2019, p. 462.
- IPCC AR6 WG1 Summary for Policymakers 2021, p. SPM-17
- IPCC AR6 WG1 Technical Summary 2021, p. TS-30.
- Rogelj et al. 2019
- ^ IPCC SR15 Summary for Policymakers 2018, p. 12
- IPCC AR5 WG3 Ch5 2014, pp. 379–380.
- Hansen et al. 2016; Smithsonian, 26 June 2016.
- USGCRP Chapter 15 2017, p. 415.
- Scientific American, 29 April 2014; Burke & Stott 2017.
- USGCRP Chapter 9 2017, p. 260.
- Studholme, Joshua; Fedorov, Alexey V.; Gulev, Sergey K.; Emanuel, Kerry; Hodges, Kevin (29 December 2021). "Poleward expansion of tropical cyclone latitudes in warming climates". Nature Geoscience. 15: 14–28. doi:10.1038/s41561-021-00859-1. S2CID 245540084.
- "Hurricanes and Climate Change". Center for Climate and Energy Solutions. 10 July 2020.
- NOAA 2017.
- WMO 2021, p. 12.
- IPCC SROCC Ch4 2019, p. 324: GMSL (global mean sea level, red) will rise between 0.43 m (0.29–0.59 m, likely range) (RCP2.6) and 0.84 m (0.61–1.10 m, likely range) (RCP8.5) by 2100 (medium confidence) relative to 1986–2005.
- DeConto & Pollard 2016.
- Bamber et al. 2019.
- Zhang et al. 2008
- IPCC SROCC Summary for Policymakers 2019, p. 18
- Doney et al. 2009.
- Deutsch et al. 2011
- IPCC SROCC Ch5 2019, p. 510; "Climate Change and Harmful Algal Blooms". EPA. 5 September 2013. Retrieved 11 September 2020.
- IPCC SR15 Ch3 2018, p. 283.
- Armstrong McKay, David I.; Staal, Arie; Abrams, Jesse F.; Winkelmann, Ricarda; Sakschewski, Boris; Loriani, Sina; Fetzer, Ingo; Cornell, Sarah E.; Rockström, Johan; Lenton, Timothy M. (9 September 2022). "Exceeding 1.5°C global warming could trigger multiple climate tipping points". Science. 377 (6611): eabn7950. doi:10.1126/science.abn7950. hdl:10871/131584. ISSN 0036-8075. PMID 36074831. S2CID 252161375.
- "Tipping points in Antarctic and Greenland ice sheets". NESSC. 12 November 2018. Retrieved 25 February 2019.
- IPCC SR15 Summary for Policymakers 2018, p. 7
- Clark et al. 2008
- Pearce, Rosamund; Prater, Tom (10 February 2020). "Nine Tipping Points That Could Be Triggered by Climate Change". CarbonBrief. Retrieved 27 May 2022.
- IPCC AR6 WG1 Summary for Policymakers 2021, p. 21
- IPCC AR5 WG1 Ch12 2013, pp. 88–89, FAQ 12.3
- IPCC AR5 WG1 Ch12 2013, p. 1112.
- Crucifix 2016
- Smith et al. 2009; Levermann et al. 2013
- IPCC SR15 Ch3 2018, p. 218.
- IPCC SRCCL Ch2 2019, p. 133.
- IPCC SRCCL Summary for Policymakers 2019, p. 7; Zeng & Yoon 2009.
- Turner et al. 2020, p. 1.
- Urban 2015.
- Poloczanska et al. 2013; Lenoir et al. 2020
- Smale et al. 2019
- IPCC SROCC Summary for Policymakers 2019, p. 13.
- IPCC SROCC Ch5 2019, p. 510
- IPCC SROCC Ch5 2019, p. 451.
- "Coral Reef Risk Outlook". National Oceanic and Atmospheric Administration. Retrieved 4 April 2020.
At present, local human activities, coupled with past thermal stress, threaten an estimated 75 percent of the world's reefs. By 2030, estimates predict more than 90% of the world's reefs will be threatened by local human activities, warming, and acidification, with nearly 60% facing high, very high, or critical threat levels.
- Carbon Brief, 7 January 2020.
- IPCC AR5 WG2 Ch28 2014, p. 1596: "Within 50 to 70 years, loss of hunting habitats may lead to elimination of polar bears from seasonally ice-covered areas, where two-thirds of their world population currently live."
- "What a changing climate means for Rocky Mountain National Park". National Park Service. Retrieved 9 April 2020.
- IPCC AR6 WG1 Summary for Policymakers 2021, Fig. SPM.6, page=SPM-23
- IPCC AR5 WG2 Ch18 2014, pp. 983, 1008
- IPCC AR5 WG2 Ch19 2014, p. 1077.
- IPCC AR5 SYR Summary for Policymakers 2014, p. 8, SPM 2
- IPCC AR5 SYR Summary for Policymakers 2014, p. 13, SPM 2.3
- WHO, Nov 2015
- IPCC AR5 WG2 Ch11 2014, pp. 720–723
- Costello et al. 2009; Watts et al. 2015; IPCC AR5 WG2 Ch11 2014, p. 713
- Watts et al. 2019, pp. 1836, 1848.
- Watts et al. 2019, pp. 1841, 1847.
- WHO 2014
- Springmann et al. 2016, p. 2; Haines & Ebi 2019
- IPCC AR6 WG2 2022, p. 988
- IPCC SRCCL Ch5 2019, p. 451.
- Zhao et al. 2017; IPCC SRCCL Ch5 2019, p. 439
- IPCC AR5 WG2 Ch7 2014, p. 488
- IPCC SRCCL Ch5 2019, p. 462
- IPCC SROCC Ch5 2019, p. 503.
- Holding et al. 2016; IPCC AR5 WG2 Ch3 2014, pp. 232–233.
- DeFries et al. 2019, p. 3; Krogstrup & Oman 2019, p. 10.
- Diffenbaugh & Burke 2019; The Guardian, 26 January 2015; Burke, Davis & Diffenbaugh 2018.
- ^ Women's leadership and gender equality in climate action and disaster risk reduction in Africa − A call for action. Accra: FAO & The African Risk Capacity (ARC) Group. 2021. doi:10.4060/cb7431en. ISBN 978-92-5-135234-2. S2CID 243488592.
- IPCC AR5 WG2 Ch13 2014, pp. 796–797
- Hallegatte et al. 2016, p. 12.
- IPCC AR5 WG2 Ch13 2014, p. 796.
- Grabe, Grose and Dutt, 2014; FAO, 2011; FAO, 2021a; Fisher and Carr, 2015; IPCC, 2014; Resurrección et al., 2019; UNDRR, 2019; Yeboah et al., 2019.
- "Climate Change | United Nations For Indigenous Peoples". United Nations Department of Economic and Social Affairs. Retrieved 29 April 2022.
- Mach et al. 2019.
- IPCC SROCC Ch4 2019, p. 328.
- UNHCR 2011, p. 3.
- Matthews 2018, p. 399.
- Balsari, Dresser & Leaning 2020
- Flavell 2014, p. 38; Kaczan & Orgill-Meyer 2020
- Serdeczny et al. 2016.
- IPCC SRCCL Ch5 2019, pp. 439, 464.
- National Oceanic and Atmospheric Administration. "What is nuisance flooding?". Retrieved 8 April 2020.
- Kabir et al. 2016.
- Van Oldenborgh et al. 2019.
- IPCC AR5 SYR Glossary 2014, p. 125.
- IPCC SR15 Summary for Policymakers 2018, p. 15
- United Nations Environment Programme 2019, p. XX
- IPCC AR6 WG3 2022, p. 300: The global benefits of pathways limiting warming to 2°C (>67%) outweigh global mitigation costs over the 21st century, if aggregated economic impacts of climate change are at the moderate to high end of the assessed range, and a weight consistent with economic theory is given to economic impacts over the long term. This holds true even without accounting for benefits in other sustainable development dimensions or nonmarket damages from climate change (medium confidence).
- IPCC SR15 Ch2 2018, p. 109.
- ^ Teske, ed. 2019, p. xxiii.
- World Resources Institute, 8 August 2019
- IPCC SR15 Ch3 2018, p. 266: Where reforestation is the restoration of natural ecosystems, it benefits both carbon sequestration and conservation of biodiversity and ecosystem services.
- Bui et al. 2018, p. 1068; IPCC SR15 Summary for Policymakers 2018, p. 17
- IPCC SR15 2018, p. 34; IPCC SR15 Summary for Policymakers 2018, p. 17
- IPCC SR15 Ch4 2018, pp. 347–352
- Friedlingstein et al. 2019
- ^ United Nations Environment Programme 2019, p. 46; Vox, 20 September 2019; Sepulveda, Nestor A.; Jenkins, Jesse D.; De Sisternes, Fernando J.; Lester, Richard K. (2018). "The Role of Firm Low-Carbon Electricity Resources in Deep Decarbonization of Power Generation". Joule. 2 (11): 2403–2420. doi:10.1016/j.joule.2018.08.006.
- REN21 2020, p. 32, Fig.1.
- Our World in Data-Why did renewables become so cheap so fast?; IEA – Projected Costs of Generating Electricity 2020
- The Guardian, 6 April 2020.
- IEA 2021, p. 57, Fig 2.5; Teske et al. 2019, p. 180, Table 8.1
- IPCC SR15 Ch2 2018, p. 131, Figure 2.15
- Teske 2019, pp. 409–410.
- United Nations Environment Programme 2019, p. XXIII, Table ES.3; Teske, ed. 2019, p. xxvii, Fig.5.
- ^ IPCC SR15 Ch2 2018, pp. 142–144; United Nations Environment Programme 2019, Table ES.3 & p. 49
- "Transport emissions". Climate action. European Commission. 2016. Archived from the original on 10 October 2021. Retrieved 2 January 2022.
- IPCC AR5 WG3 Ch9 2014, p. 697; NREL 2017, pp. vi, 12
- Berrill et al. 2016.
- IPCC SR15 Ch4 2018, pp. 324–325.
- Gill, Matthew; Livens, Francis; Peakman, Aiden. "Nuclear Fission". In Letcher (2020), pp. 147–149.
- Horvath, Akos; Rachlew, Elisabeth (January 2016). "Nuclear power in the 21st century: Challenges and possibilities". Ambio. 45 (Suppl 1): S38–49. doi:10.1007/s13280-015-0732-y. ISSN 1654-7209. PMC 4678124. PMID 26667059.
- "Hydropower". iea.org. International Energy Agency. Retrieved 12 October 2020.
Hydropower generation is estimated to have increased by over 2% in 2019 owing to continued recovery from drought in Latin America as well as strong capacity expansion and good water availability in China (...) capacity expansion has been losing speed. This downward trend is expected to continue, due mainly to less large-project development in China and Brazil, where concerns over social and environmental impacts have restricted projects.
- Watts et al. 2019, p. 1854; WHO 2018, p. 27
- Watts et al. 2019, p. 1837; WHO 2016
- WHO 2018, p. 27; Vandyck et al. 2018; IPCC SR15 2018, p. 97: "Limiting warming to 1.5 °C can be achieved synergistically with poverty alleviation and improved energy security and can provide large public health benefits through improved air quality, preventing millions of premature deaths. However, specific mitigation measures, such as bioenergy, may result in trade-offs that require consideration."
- IPCC AR6 WG3 2022, p. 300
- IPCC SR15 Ch2 2018, p. 97
- IPCC AR5 SYR Summary for Policymakers 2014, p. 29; IEA 2020b
- IPCC SR15 Ch2 2018, p. 155, Fig. 2.27
- IEA 2020b
- IPCC SR15 Ch2 2018, p. 142
- IPCC SR15 Ch2 2018, pp. 138–140
- IPCC SR15 Ch2 2018, pp. 141–142
- IPCC AR5 WG3 Ch9 2014, pp. 686–694.
- World Resources Institute, December 2019, p. 1
- World Resources Institute, December 2019, pp. 1, 3
- IPCC SRCCL 2019, p. 22, B.6.2
- IPCC SRCCL Ch5 2019, pp. 487, 488, FIGURE 5.12 Humans on a vegan exclusive diet would save about 7.9 GtCO2 equivalent per year by 2050 IPCC AR6 WG1 Technical Summary 2021, p. 51 Agriculture, Forestry and Other Land Use used an average of 12 GtCO2 per year between 2007 and 2016 (23% of total anthropogenic emissions).
- IPCC SRCCL Ch5 2019, pp. 82, 162, FIGURE 1.1
- "Low and zero emissions in the steel and cement industries" (PDF). pp. 11, 19–22.
- World Resources Institute, 8 August 2019: IPCC SRCCL Ch2 2019, pp. 189–193.
- Kreidenweis et al. 2016
- National Academies of Sciences, Engineering, and Medicine 2019, pp. 95–102
- National Academies of Sciences, Engineering, and Medicine 2019, pp. 45–54
- Ruseva et al. 2020
- IPCC SR15 Ch4 2018, pp. 326–327; Bednar, Obersteiner & Wagner 2019; European Commission, 28 November 2018, p. 188
- Bui et al. 2018, p. 1068.
- IPCC AR5 SYR 2014, p. 125; Bednar, Obersteiner & Wagner 2019.
- IPCC SR15 2018, p. 34
- IPCC, 2022: Summary for Policymakers . In: Climate Change 2022: Impacts, Adaptation and Vulnerability. Contribution of Working Group II to the Sixth Assessment Report of the Intergovernmental Panel on Climate Change . Cambridge University Press, Cambridge, UK and New York, NY, USA, pp. 3–33, doi:10.1017/9781009325844.001.
- IPCC AR5 SYR 2014, p. 17.
- IPCC SR15 Ch4 2018, pp. 396–397.
- IPCC AR4 WG2 Ch19 2007, p. 796.
- UNEP 2018, pp. xii–xiii.
- Stephens, Scott A.; Bell, Robert G.; Lawrence, Judy (2018). "Developing signals to trigger adaptation to sea-level rise". Environmental Research Letters. 13 (10). 104004. Bibcode:2018ERL....13j4004S. doi:10.1088/1748-9326/aadf96. ISSN 1748-9326.
- Matthews 2018, p. 402.
- IPCC SRCCL Ch5 2019, p. 439.
- Surminski, Swenja; Bouwer, Laurens M.; Linnerooth-Bayer, Joanne (2016). "How insurance can support climate resilience". Nature Climate Change. 6 (4): 333–334. Bibcode:2016NatCC...6..333S. doi:10.1038/nclimate2979. ISSN 1758-6798.
- IPCC SR15 Ch4 2018, pp. 336–337.
- "Mangroves against the storm". Shorthand. Retrieved 20 January 2023.
- "How marsh grass could help protect us from climate change". World Economic Forum. Retrieved 20 January 2023.
- Morecroft, Michael D.; Duffield, Simon; Harley, Mike; Pearce-Higgins, James W.; et al. (2019). "Measuring the success of climate change adaptation and mitigation in terrestrial ecosystems". Science. 366 (6471): eaaw9256. doi:10.1126/science.aaw9256. ISSN 0036-8075. PMID 31831643. S2CID 209339286.
- Berry, Pam M.; Brown, Sally; Chen, Minpeng; Kontogianni, Areti; et al. (2015). "Cross-sectoral interactions of adaptation and mitigation measures". Climate Change. 128 (3): 381–393. Bibcode:2015ClCh..128..381B. doi:10.1007/s10584-014-1214-0. ISSN 1573-1480. S2CID 153904466.
- Sharifi, Ayyoob (2020). "Trade-offs and conflicts between urban climate change mitigation and adaptation measures: A literature review". Journal of Cleaner Production. 276: 122813. doi:10.1016/j.jclepro.2020.122813. ISSN 0959-6526. S2CID 225638176.
- IPCC AR5 SYR 2014, p. 54.
- IPCC AR5 SYR Summary for Policymakers 2014, p. 17, Section 3
- IPCC SR15 Ch5 2018, p. 447; United Nations (2017) Resolution adopted by the General Assembly on 6 July 2017, Work of the Statistical Commission pertaining to the 2030 Agenda for Sustainable Development (A/RES/71/313)
- IPCC SR15 Ch5 2018, p. 477.
- Rauner et al. 2020
- Mercure et al. 2018
- World Bank, June 2019, p. 12, Box 1
- Union of Concerned Scientists, 8 January 2017; Hagmann, Ho & Loewenstein 2019.
- Watts et al. 2019, p. 1866
- UN Human Development Report 2020, p. 10
- International Institute for Sustainable Development 2019, p. iv
- ICCT 2019, p. iv; Natural Resources Defense Council, 29 September 2017
- National Conference of State Legislators, 17 April 2020; European Parliament, February 2020
- Gabbatiss, Josh; Tandon, Ayesha (4 October 2021). "In-depth Q&A: What is 'climate justice'?". Carbon Brief. Retrieved 16 October 2021.
- Carbon Brief, 4 Jan 2017.
- ^ Friedlingstein et al. 2019, Table 7.
- UNFCCC, "What is the United Nations Framework Convention on Climate Change?"
- UNFCCC 1992, Article 2.
- IPCC AR4 WG3 Ch1 2007, p. 97.
- EPA 2019.
- UNFCCC, "What are United Nations Climate Change Conferences?"
- Kyoto Protocol 1997; Liverman 2009, p. 290.
- Dessai 2001, p. 4; Grubb 2003.
- Liverman 2009, p. 290.
- Müller 2010; The New York Times, 25 May 2015; UNFCCC: Copenhagen 2009; EUobserver, 20 December 2009.
- UNFCCC: Copenhagen 2009.
- Conference of the Parties to the Framework Convention on Climate Change. Copenhagen. 7–18 December 2009. un document= FCCC/CP/2009/L.7. Archived from the original on 18 October 2010. Retrieved 24 October 2010.
- Cui, Lianbiao; Sun, Yi; Song, Malin; Zhu, Lei (2020). "Co-financing in the green climate fund: lessons from the global environment facility". Climate Policy. 20 (1): 95–108. doi:10.1080/14693062.2019.1690968. ISSN 1469-3062. S2CID 213694904.
- Paris Agreement 2015.
- Climate Focus 2015, p. 3; Carbon Brief, 8 October 2018.
- Climate Focus 2015, p. 5.
- "Status of Treaties, United Nations Framework Convention on Climate Change". United Nations Treaty Collection. Retrieved 13 October 2021.; Salon, 25 September 2019.
- Goyal et al. 2019
- Yeo, Sophie (10 October 2016). "Explainer: Why a UN climate deal on HFCs matters". Carbon Brief. Retrieved 10 January 2021.
- BBC, 1 May 2019; Vice, 2 May 2019.
- The Verge, 27 December 2019.
- The Guardian, 28 November 2019
- Politico, 11 December 2019.
- The Guardian, 28 October 2020
- "European Green Deal: Commission proposes transformation of EU economy and society to meet climate ambitions". European Commission. 14 July 2021.
- "India". Climate Action Tracker. 15 September 2021. Retrieved 3 October 2021.
- Do, Thang Nam; Burke, Paul J. "Phasing out coal power in a developing country context: Insights from Vietnam". Energy Policy. 176 (May 2023 113512). doi:10.1016/j.enpol.2023.113512.
- UN NDC Synthesis Report 2021, pp. 4–5; UNFCCC Press Office (26 February 2021). "Greater Climate Ambition Urged as Initial NDC Synthesis Report Is Published". Retrieved 21 April 2021.
- Stover 2014.
- Dunlap & McCright 2011, pp. 144, 155; Björnberg et al. 2017
- Oreskes & Conway 2010; Björnberg et al. 2017
- O’Neill & Boykoff 2010; Björnberg et al. 2017
- ^ Björnberg et al. 2017
- Dunlap & McCright 2015, p. 308.
- Dunlap & McCright 2011, p. 146.
- Harvey et al. 2018
- "Public perceptions on climate change" (PDF). PERITIA Trust EU - The Policy Institute of Kings College London. June 2022. p. 4. Archived (PDF) from the original on 15 July 2022.
- Powell, James (20 November 2019). "Scientists Reach 100% Consensus on Anthropogenic Global Warming". Bulletin of Science, Technology & Society. 37 (4): 183–184. doi:10.1177/0270467619886266. S2CID 213454806.
- Myers, Krista F.; Doran, Peter T.; Cook, John; Kotcher, John E.; Myers, Teresa A. (20 October 2021). "Consensus revisited: quantifying scientific agreement on climate change and climate expertise among Earth scientists 10 years later". Environmental Research Letters. 16 (10): 104030. Bibcode:2021ERL....16j4030M. doi:10.1088/1748-9326/ac2774. S2CID 239047650.
- ^ Weart "The Public and Climate Change (since 1980)"
- Newell 2006, p. 80; Yale Climate Connections, 2 November 2010
- Pew 2015, p. 10.
- ^ Pew 2020.
- Pew 2015, p. 15.
- Yale 2021, p. 7.
- Yale 2021, p. 9; UNDP 2021, p. 15.
- Smith & Leiserowitz 2013, p. 943.
- Gunningham 2018.
- The Guardian, 19 March 2019; Boulianne, Lalancette & Ilkiw 2020.
- Deutsche Welle, 22 June 2019.
- Connolly, Kate (29 April 2021). "'Historic' German ruling says climate goals not tough enough". The Guardian. Retrieved 1 May 2021.
- Setzer & Byrnes 2019.
- "Coal Consumption Affecting Climate". Rodney and Otamatea Times, Waitemata and Kaipara Gazette. Warkworth, New Zealand. 14 August 1912. p. 7. Text was earlier published in Popular Mechanics, March 1912, p. 341.
- Nord, D.C. (2020). Nordic Perspectives on the Responsible Development of the Arctic: Pathways to Action. Springer Polar Sciences. Springer International Publishing. p. 51. ISBN 978-3-030-52324-4. Retrieved 11 March 2023.
- Mukherjee, A.; Scanlon, B.R.; Aureli, A.; Langan, S.; Guo, H.; McKenzie, A.A. (2020). Global Groundwater: Source, Scarcity, Sustainability, Security, and Solutions. Elsevier Science. p. 331. ISBN 978-0-12-818173-7. Retrieved 11 March 2023.
- von Humboldt, A.; Wulf, A. (2018). Selected Writings of Alexander von Humboldt: Edited and Introduced by Andrea Wulf. Everyman's Library Classics Series. Knopf Doubleday Publishing Group. p. 10. ISBN 978-1-101-90807-5. Retrieved 11 March 2023.
- Erdkamp, P.; Manning, J.G.; Verboven, K. (2021). Climate Change and Ancient Societies in Europe and the Near East: Diversity in Collapse and Resilience. Palgrave Studies in Ancient Economies. Springer International Publishing. p. 6. ISBN 978-3-030-81103-7. Retrieved 11 March 2023.
- Archer & Pierrehumbert 2013, pp. 10–14
- Foote, Eunice (November 1856). Circumstances affecting the Heat of the Sun's Rays. Vol. 22. pp. 382–383. Retrieved 31 January 2016 – via Google Books.
{{cite book}}
:|work=
ignored (help) - Huddleston 2019
- Tyndall 1861.
- Archer & Pierrehumbert 2013, pp. 39–42; Fleming 2008, Tyndall
- Lapenis 1998.
- ^ Weart "The Carbon Dioxide Greenhouse Effect"; Fleming 2008, Arrhenius
- Callendar 1938; Fleming 2007.
- Weart "Suspicions of a Human-Caused Greenhouse (1956–1969)"
- Weart 2013, p. 3567.
- Royal Society 2005.
- Powell, James (20 November 2019). "Scientists Reach 100% Consensus on Anthropogenic Global Warming". Bulletin of Science, Technology & Society. 37 (4): 183–184. doi:10.1177/0270467619886266. S2CID 213454806. Retrieved 15 November 2020.
- ^ Lynas, Mark; Houlton, Benjamin Z; Perry, Simon (2021). "Greater than 99% consensus on human caused climate change in the peer-reviewed scientific literature". Environmental Research Letters. 16 (11): 114005. Bibcode:2021ERL....16k4005L. doi:10.1088/1748-9326/ac2966. ISSN 1748-9326. S2CID 239032360.
- National Academies 2008, p. 2; Oreskes 2007, p. 68; Gleick, 7 January 2017
- Joint statement of the G8+5 Academies (2009); Gleick, 7 January 2017.
Sources
IPCC reports
Fourth Assessment Report
- IPCC (2007). Solomon, S.; Qin, D.; Manning, M.; Chen, Z.; et al. (eds.). Climate Change 2007: The Physical Science Basis. Contribution of Working Group I to the Fourth Assessment Report of the Intergovernmental Panel on Climate Change. Cambridge University Press. ISBN 978-0-521-88009-1.
- Le Treut, H.; Somerville, R.; Cubasch, U.; Ding, Y.; et al. (2007). "Chapter 1: Historical Overview of Climate Change Science" (PDF). IPCC AR4 WG1 2007. pp. 93–127.
- Randall, D. A.; Wood, R. A.; Bony, S.; Colman, R.; et al. (2007). "Chapter 8: Climate Models and their Evaluation" (PDF). IPCC AR4 WG1 2007. pp. 589–662.
- Hegerl, G. C.; Zwiers, F. W.; Braconnot, P.; Gillett, N. P.; et al. (2007). "Chapter 9: Understanding and Attributing Climate Change" (PDF). IPCC AR4 WG1 2007. pp. 663–745.
- IPCC (2007). Parry, M. L.; Canziani, O. F.; Palutikof, J. P.; van der Linden, P. J.; et al. (eds.). Climate Change 2007: Impacts, Adaptation and Vulnerability. Contribution of Working Group II to the Fourth Assessment Report of the Intergovernmental Panel on Climate Change. Cambridge University Press. ISBN 978-0-521-88010-7.
- Rosenzweig, C.; Casassa, G.; Karoly, D. J.; Imeson, A.; et al. (2007). "Chapter 1: Assessment of observed changes and responses in natural and managed systems" (PDF). IPCC AR4 WG2 2007. pp. 79–131.
- Schneider, S. H.; Semenov, S.; Patwardhan, A.; Burton, I.; et al. (2007). "Chapter 19: Assessing key vulnerabilities and the risk from climate change" (PDF). IPCC AR4 WG2 2007. pp. 779–810.
- IPCC (2007). Metz, B.; Davidson, O. R.; Bosch, P. R.; Dave, R.; et al. (eds.). Climate Change 2007: Mitigation of Climate Change. Contribution of Working Group III to the Fourth Assessment Report of the Intergovernmental Panel on Climate Change. Cambridge University Press. ISBN 978-0-521-88011-4.
- Rogner, H.-H.; Zhou, D.; Bradley, R.; Crabbé, P.; et al. (2007). "Chapter 1: Introduction" (PDF). IPCC AR4 WG3 2007. pp. 95–116.
Fifth Assessment report
- IPCC (2013). Stocker, T. F.; Qin, D.; Plattner, G.-K.; Tignor, M.; et al. (eds.). Climate Change 2013: The Physical Science Basis (PDF). Contribution of Working Group I to the Fifth Assessment Report of the Intergovernmental Panel on Climate Change. Cambridge, UK & New York: Cambridge University Press. ISBN 978-1-107-05799-9.. AR5 Climate Change 2013: The Physical Science Basis — IPCC
- IPCC (2013). "Summary for Policymakers" (PDF). IPCC AR5 WG1 2013.
- Hartmann, D. L.; Klein Tank, A. M. G.; Rusticucci, M.; Alexander, L. V.; et al. (2013). "Chapter 2: Observations: Atmosphere and Surface" (PDF). IPCC AR5 WG1 2013. pp. 159–254.
- Rhein, M.; Rintoul, S. R.; Aoki, S.; Campos, E.; et al. (2013). "Chapter 3: Observations: Ocean" (PDF). IPCC AR5 WG1 2013. pp. 255–315.
- Masson-Delmotte, V.; Schulz, M.; Abe-Ouchi, A.; Beer, J.; et al. (2013). "Chapter 5: Information from Paleoclimate Archives" (PDF). IPCC AR5 WG1 2013. pp. 383–464.
- Bindoff, N. L.; Stott, P. A.; AchutaRao, K. M.; Allen, M. R.; et al. (2013). "Chapter 10: Detection and Attribution of Climate Change: from Global to Regional" (PDF). IPCC AR5 WG1 2013. pp. 867–952.
- Collins, M.; Knutti, R.; Arblaster, J. M.; Dufresne, J.-L.; et al. (2013). "Chapter 12: Long-term Climate Change: Projections, Commitments and Irreversibility" (PDF). IPCC AR5 WG1 2013. pp. 1029–1136.
- IPCC (2014). Field, C. B.; Barros, V. R.; Dokken, D. J.; Mach, K. J.; et al. (eds.). Climate Change 2014: Impacts, Adaptation, and Vulnerability. Part A: Global and Sectoral Aspects. Contribution of Working Group II to the Fifth Assessment Report of the Intergovernmental Panel on Climate Change. Cambridge University Press. ISBN 978-1-107-05807-1.. Chapters 1–20, SPM, and Technical Summary.
- Jiménez Cisneros, B. E.; Oki, T.; Arnell, N. W.; Benito, G.; et al. (2014). "Chapter 3: Freshwater Resources" (PDF). IPCC AR5 WG2 A 2014. pp. 229–269.
- Porter, J. R.; Xie, L.; Challinor, A. J.; Cochrane, K.; et al. (2014). "Chapter 7: Food Security and Food Production Systems" (PDF). IPCC AR5 WG2 A 2014. pp. 485–533.
- Smith, K. R.; Woodward, A.; Campbell-Lendrum, D.; Chadee, D. D.; et al. (2014). "Chapter 11: Human Health: Impacts, Adaptation, and Co-Benefits" (PDF). In IPCC AR5 WG2 A 2014. pp. 709–754.
- Olsson, L.; Opondo, M.; Tschakert, P.; Agrawal, A.; et al. (2014). "Chapter 13: Livelihoods and Poverty" (PDF). IPCC AR5 WG2 A 2014. pp. 793–832.
- Cramer, W.; Yohe, G. W.; Auffhammer, M.; Huggel, C.; et al. (2014). "Chapter 18: Detection and Attribution of Observed Impacts" (PDF). IPCC AR5 WG2 A 2014. pp. 979–1037.
- Oppenheimer, M.; Campos, M.; Warren, R.; Birkmann, J.; et al. (2014). "Chapter 19: Emergent Risks and Key Vulnerabilities" (PDF). IPCC AR5 WG2 A 2014. pp. 1039–1099.
- IPCC (2014). Barros, V. R.; Field, C. B.; Dokken, D. J.; Mach, K. J.; et al. (eds.). Climate Change 2014: Impacts, Adaptation, and Vulnerability. Part B: Regional Aspects (PDF). Contribution of Working Group II to the Fifth Assessment Report of the Intergovernmental Panel on Climate Change. Cambridge, UK & New York: Cambridge University Press. ISBN 978-1-107-05816-3.. Chapters 21–30, Annexes, and Index.
- Larsen, J. N.; Anisimov, O. A.; Constable, A.; Hollowed, A. B.; et al. (2014). "Chapter 28: Polar Regions" (PDF). IPCC AR5 WG2 B 2014. pp. 1567–1612.
- IPCC (2014). Edenhofer, O.; Pichs-Madruga, R.; Sokona, Y.; Farahani, E.; et al. (eds.). Climate Change 2014: Mitigation of Climate Change. Contribution of Working Group III to the Fifth Assessment Report of the Intergovernmental Panel on Climate Change. Cambridge, UK & New York, NY: Cambridge University Press. ISBN 978-1-107-05821-7.
- Blanco, G.; Gerlagh, R.; Suh, S.; Barrett, J.; et al. (2014). "Chapter 5: Drivers, Trends and Mitigation" (PDF). IPCC AR5 WG3 2014. pp. 351–411.
- Lucon, O.; Ürge-Vorsatz, D.; Ahmed, A.; Akbari, H.; et al. (2014). "Chapter 9: Buildings" (PDF). IPCC AR5 WG3 2014.
- IPCC AR5 SYR (2014). The Core Writing Team; Pachauri, R. K.; Meyer, L. A. (eds.). Climate Change 2014: Synthesis Report. Contribution of Working Groups I, II and III to the Fifth Assessment Report of the Intergovernmental Panel on Climate Change. Geneva, Switzerland: IPCC.
{{cite book}}
: CS1 maint: numeric names: authors list (link)- IPCC (2014). "Summary for Policymakers" (PDF). IPCC AR5 SYR 2014.
- IPCC (2014). "Annex II: Glossary" (PDF). IPCC AR5 SYR 2014.
Special Report: Global Warming of 1.5 °C
- IPCC (2018). Masson-Delmotte, V.; Zhai, P.; Pörtner, H.-O.; Roberts, D.; et al. (eds.). Global Warming of 1.5°C. An IPCC Special Report on the impacts of global warming of 1.5°C above pre-industrial levels and related global greenhouse gas emission pathways, in the context of strengthening the global response to the threat of climate change, sustainable development, and efforts to eradicate poverty (PDF). Intergovernmental Panel on Climate Change. Global Warming of 1.5 ºC —.
- IPCC (2018). "Summary for Policymakers" (PDF). IPCC SR15 2018. pp. 3–24.
- Allen, M. R.; Dube, O. P.; Solecki, W.; Aragón-Durand, F.; et al. (2018). "Chapter 1: Framing and Context" (PDF). IPCC SR15 2018. pp. 49–91.
- Rogelj, J.; Shindell, D.; Jiang, K.; Fifta, S.; et al. (2018). "Chapter 2: Mitigation Pathways Compatible with 1.5°C in the Context of Sustainable Development" (PDF). IPCC SR15 2018. pp. 93–174.
- Hoegh-Guldberg, O.; Jacob, D.; Taylor, M.; Bindi, M.; et al. (2018). "Chapter 3: Impacts of 1.5ºC Global Warming on Natural and Human Systems" (PDF). IPCC SR15 2018. pp. 175–311.
- de Coninck, H.; Revi, A.; Babiker, M.; Bertoldi, P.; et al. (2018). "Chapter 4: Strengthening and Implementing the Global Response" (PDF). IPCC SR15 2018. pp. 313–443.
- Roy, J.; Tschakert, P.; Waisman, H.; Abdul Halim, S.; et al. (2018). "Chapter 5: Sustainable Development, Poverty Eradication and Reducing Inequalities" (PDF). IPCC SR15 2018. pp. 445–538.
Special Report: Climate change and Land
- IPCC (2019). Shukla, P. R.; Skea, J.; Calvo Buendia, E.; Masson-Delmotte, V.; et al. (eds.). IPCC Special Report on Climate Change, Desertification, Land Degradation, Sustainable Land Management, Food Security, and Greenhouse gas fluxes in Terrestrial Ecosystems (PDF). In press.
- IPCC (2019). "Summary for Policymakers" (PDF). IPCC SRCCL 2019. pp. 3–34.
- Jia, G.; Shevliakova, E.; Artaxo, P. E.; De Noblet-Ducoudré, N.; et al. (2019). "Chapter 2: Land-Climate Interactions" (PDF). IPCC SRCCL 2019. pp. 131–247.
- Mbow, C.; Rosenzweig, C.; Barioni, L. G.; Benton, T.; et al. (2019). "Chapter 5: Food Security" (PDF). IPCC SRCCL 2019. pp. 437–550.
Special Report: The Ocean and Cryosphere in a Changing Climate
- IPCC (2019). Pörtner, H.-O.; Roberts, D. C.; Masson-Delmotte, V.; Zhai, P.; et al. (eds.). IPCC Special Report on the Ocean and Cryosphere in a Changing Climate (PDF). In press.
- IPCC (2019). "Summary for Policymakers" (PDF). IPCC SROCC 2019. pp. 3–35.
- Meredith, M.; Sommerkorn, M.; Cassotta, S.; Derksen, C.; et al. (2019). "Chapter 3: Polar Regions" (PDF). IPCC SROCC 2019. pp. 203–320.
- Oppenheimer, M.; Glavovic, B.; Hinkel, J.; van de Wal, R.; et al. (2019). "Chapter 4: Sea Level Rise and Implications for Low Lying Islands, Coasts and Communities" (PDF). IPCC SROCC 2019. pp. 321–445.
- Bindoff, N. L.; Cheung, W. W. L.; Kairo, J. G.; Arístegui, J.; et al. (2019). "Chapter 5: Changing Ocean, Marine Ecosystems, and Dependent Communities" (PDF). IPCC SROCC 2019. pp. 447–587.
Sixth Assessment Report
- IPCC (2021). Masson-Delmotte, V.; Zhai, P.; Pirani, A.; Connors, S. L.; et al. (eds.). Climate Change 2021: The Physical Science Basis (PDF). Contribution of Working Group I to the Sixth Assessment Report of the Intergovernmental Panel on Climate Change. Cambridge, United Kingdom and New York, NY, USA: Cambridge University Press (In Press).
- IPCC (2021). "Summary for Policymakers" (PDF). IPCC AR6 WG1 2021.
- Arias, Paola A.; Bellouin, Nicolas; Coppola, Erika; Jones, Richard G.; et al. (2021). "Technical Summary" (PDF). IPCC AR6 WG1 2021.
- Seneviratne, Sonia I.; Zhang, Xuebin; Adnan, M.; Badi, W.; et al. (2021). "Chapter 11: Weather and climate extreme events in a changing climate" (PDF). IPCC AR6 WG1 2021.
- IPCC (2022). Pörtner, H.-O.; Roberts, D.C.; Tignor, M.; Poloczanska, E.S.; Mintenbeck, K.; Alegría, A.; Craig, M.; Langsdorf, S.; Löschke, S.; Möller, V.; Okem, A.; Rama, B.; et al. (eds.). Climate Change 2022: Impacts, Adaptation and Vulnerability. Contribution of Working Group II to the Sixth Assessment Report of the Intergovernmental Panel on Climate Change. Cambridge University Press.
- IPCC (2022). Shukla, P.R.; Skea, J.; Slade, R.; Al Khourdajie, A.; et al. (eds.). Climate Change 2022: Mitigation of Climate Change. Contribution of Working Group III to the Sixth Assessment Report of the Intergovernmental Panel on Climate Change. Cambridge University Press.
- IPCC (2022). "Summary for Policymakers" (PDF). IPCC AR6 WG3 2022.
Other peer-reviewed sources
- Albrecht, Bruce A. (1989). "Aerosols, Cloud Microphysics, and Fractional Cloudiness". Science. 245 (4923): 1227–1239. Bibcode:1989Sci...245.1227A. doi:10.1126/science.245.4923.1227. PMID 17747885. S2CID 46152332.
- Balsari, S.; Dresser, C.; Leaning, J. (2020). "Climate Change, Migration, and Civil Strife". Curr Environ Health Rep. 7 (4): 404–414. doi:10.1007/s40572-020-00291-4. PMC 7550406. PMID 33048318.
- Bamber, Jonathan L.; Oppenheimer, Michael; Kopp, Robert E.; Aspinall, Willy P.; Cooke, Roger M. (2019). "Ice sheet contributions to future sea-level rise from structured expert judgment". Proceedings of the National Academy of Sciences. 116 (23): 11195–11200. Bibcode:2019PNAS..11611195B. doi:10.1073/pnas.1817205116. ISSN 0027-8424. PMC 6561295. PMID 31110015.
- Bednar, Johannes; Obersteiner, Michael; Wagner, Fabian (2019). "On the financial viability of negative emissions". Nature Communications. 10 (1): 1783. Bibcode:2019NatCo..10.1783B. doi:10.1038/s41467-019-09782-x. ISSN 2041-1723. PMC 6467865. PMID 30992434.
- Berrill, P.; Arvesen, A.; Scholz, Y.; Gils, H. C.; et al. (2016). "Environmental impacts of high penetration renewable energy scenarios for Europe". Environmental Research Letters. 11 (1): 014012. Bibcode:2016ERL....11a4012B. doi:10.1088/1748-9326/11/1/014012.
- Björnberg, Karin Edvardsson; Karlsson, Mikael; Gilek, Michael; Hansson, Sven Ove (2017). "Climate and environmental science denial: A review of the scientific literature published in 1990–2015". Journal of Cleaner Production. 167: 229–241. doi:10.1016/j.jclepro.2017.08.066. ISSN 0959-6526.
- Boulianne, Shelley; Lalancette, Mireille; Ilkiw, David (2020). ""School Strike 4 Climate": Social Media and the International Youth Protest on Climate Change". Media and Communication. 8 (2): 208–218. doi:10.17645/mac.v8i2.2768. ISSN 2183-2439.
- Bui, M.; Adjiman, C.; Bardow, A.; Anthony, Edward J.; et al. (2018). "Carbon capture and storage (CCS): the way forward". Energy & Environmental Science. 11 (5): 1062–1176. doi:10.1039/c7ee02342a.
- Burke, Claire; Stott, Peter (2017). "Impact of Anthropogenic Climate Change on the East Asian Summer Monsoon". Journal of Climate. 30 (14): 5205–5220. arXiv:1704.00563. Bibcode:2017JCli...30.5205B. doi:10.1175/JCLI-D-16-0892.1. ISSN 0894-8755. S2CID 59509210.
- Burke, Marshall; Davis, W. Matthew; Diffenbaugh, Noah S (2018). "Large potential reduction in economic damages under UN mitigation targets". Nature. 557 (7706): 549–553. Bibcode:2018Natur.557..549B. doi:10.1038/s41586-018-0071-9. ISSN 1476-4687. PMID 29795251. S2CID 43936274.
- Callendar, G. S. (1938). "The artificial production of carbon dioxide and its influence on temperature". Quarterly Journal of the Royal Meteorological Society. 64 (275): 223–240. Bibcode:1938QJRMS..64..223C. doi:10.1002/qj.49706427503.
- Cattaneo, Cristina; Beine, Michel; Fröhlich, Christiane J.; Kniveton, Dominic; et al. (2019). "Human Migration in the Era of Climate Change". Review of Environmental Economics and Policy. 13 (2): 189–206. doi:10.1093/reep/rez008. hdl:10.1093/reep/rez008. ISSN 1750-6816. S2CID 198660593.
- Cohen, Judah; Screen, James; Furtado, Jason C.; Barlow, Mathew; et al. (2014). "Recent Arctic amplification and extreme mid-latitude weather" (PDF). Nature Geoscience. 7 (9): 627–637. Bibcode:2014NatGe...7..627C. doi:10.1038/ngeo2234. ISSN 1752-0908.
- Costello, Anthony; Abbas, Mustafa; Allen, Adriana; Ball, Sarah; et al. (2009). "Managing the health effects of climate change". The Lancet. 373 (9676): 1693–1733. doi:10.1016/S0140-6736(09)60935-1. PMID 19447250. S2CID 205954939. Archived from the original on 13 August 2017.
- Curtis, P.; Slay, C.; Harris, N.; Tyukavina, A.; et al. (2018). "Classifying drivers of global forest loss". Science. 361 (6407): 1108–1111. Bibcode:2018Sci...361.1108C. doi:10.1126/science.aau3445. PMID 30213911. S2CID 52273353.
- Davidson, Eric (2009). "The contribution of manure and fertilizer nitrogen to atmospheric nitrous oxide since 1860". Nature Geoscience. 2: 659–662. doi:10.1016/j.chemer.2016.04.002.
- DeConto, Robert M.; Pollard, David (2016). "Contribution of Antarctica to past and future sea-level rise". Nature. 531 (7596): 591–597. Bibcode:2016Natur.531..591D. doi:10.1038/nature17145. ISSN 1476-4687. PMID 27029274. S2CID 205247890.
- Dean, Joshua F.; Middelburg, Jack J.; Röckmann, Thomas; Aerts, Rien; et al. (2018). "Methane Feedbacks to the Global Climate System in a Warmer World". Reviews of Geophysics. 56 (1): 207–250. Bibcode:2018RvGeo..56..207D. doi:10.1002/2017RG000559. ISSN 1944-9208.
- Delworth, Thomas L.; Zeng, Fanrong (2012). "Multicentennial variability of the Atlantic meridional overturning circulation and its climatic influence in a 4000 year simulation of the GFDL CM2.1 climate model". Geophysical Research Letters. 39 (13): n/a. Bibcode:2012GeoRL..3913702D. doi:10.1029/2012GL052107. ISSN 1944-8007.
- Deutsch, Curtis; Brix, Holger; Ito, Taka; Frenzel, Hartmut; et al. (2011). "Climate-Forced Variability of Ocean Hypoxia" (PDF). Science. 333 (6040): 336–339. Bibcode:2011Sci...333..336D. doi:10.1126/science.1202422. PMID 21659566. S2CID 11752699. Archived (PDF) from the original on 9 May 2016.
- Diffenbaugh, Noah S.; Burke, Marshall (2019). "Global warming has increased global economic inequality". Proceedings of the National Academy of Sciences. 116 (20): 9808–9813. Bibcode:2019PNAS..116.9808D. doi:10.1073/pnas.1816020116. ISSN 0027-8424. PMC 6525504. PMID 31010922.
- Doney, Scott C.; Fabry, Victoria J.; Feely, Richard A.; Kleypas, Joan A. (2009). "Ocean Acidification: The Other CO2 Problem". Annual Review of Marine Science. 1 (1): 169–192. Bibcode:2009ARMS....1..169D. doi:10.1146/annurev.marine.010908.163834. PMID 21141034. S2CID 402398.
- Fahey, D. W.; Doherty, S. J.; Hibbard, K. A.; Romanou, A.; Taylor, P. C. (2017). "Chapter 2: Physical Drivers of Climate Change" (PDF). In USGCRP2017.
- Fischer, Tobias P.; Aiuppa, Alessandro (2020). "AGU Centennial Grand Challenge: Volcanoes and Deep Carbon Global CO2 Emissions From Subaerial Volcanism – Recent Progress and Future Challenges". Geochemistry, Geophysics, Geosystems. 21 (3): e08690. Bibcode:2020GGG....2108690F. doi:10.1029/2019GC008690. ISSN 1525-2027.
- Franzke, Christian L. E.; Barbosa, Susana; Blender, Richard; Fredriksen, Hege-Beate; et al. (2020). "The Structure of Climate Variability Across Scales". Reviews of Geophysics. 58 (2): e2019RG000657. Bibcode:2020RvGeo..5800657F. doi:10.1029/2019RG000657. ISSN 1944-9208.
- Friedlingstein, Pierre; Jones, Matthew W.; O'Sullivan, Michael; Andrew, Robbie M.; et al. (2019). "Global Carbon Budget 2019". Earth System Science Data. 11 (4): 1783–1838. Bibcode:2019ESSD...11.1783F. doi:10.5194/essd-11-1783-2019. ISSN 1866-3508.
- Fyfe, John C.; Meehl, Gerald A.; England, Matthew H.; Mann, Michael E.; et al. (2016). "Making sense of the early-2000s warming slowdown" (PDF). Nature Climate Change. 6 (3): 224–228. Bibcode:2016NatCC...6..224F. doi:10.1038/nclimate2938. S2CID 52474791. Archived (PDF) from the original on 7 February 2019.
- Goyal, Rishav; England, Matthew H; Sen Gupta, Alex; Jucker, Martin (2019). "Reduction in surface climate change achieved by the 1987 Montreal Protocol". Environmental Research Letters. 14 (12): 124041. Bibcode:2019ERL....14l4041G. doi:10.1088/1748-9326/ab4874. ISSN 1748-9326.
- Grubb, M. (2003). "The Economics of the Kyoto Protocol" (PDF). World Economics. 4 (3): 144–145. Archived from the original (PDF) on 4 September 2012.
- Gunningham, Neil (2018). "Mobilising civil society: can the climate movement achieve transformational social change?" (PDF). Interface: A Journal for and About Social Movements. 10. Archived (PDF) from the original on 12 April 2019. Retrieved 12 April 2019.
- Hagmann, David; Ho, Emily H.; Loewenstein, George (2019). "Nudging out support for a carbon tax". Nature Climate Change. 9 (6): 484–489. Bibcode:2019NatCC...9..484H. doi:10.1038/s41558-019-0474-0. S2CID 182663891.
- Haines, A.; Ebi, K. (2019). "The Imperative for Climate Action to Protect Health". New England Journal of Medicine. 380 (3): 263–273. doi:10.1056/NEJMra1807873. PMID 30650330. S2CID 58662802.
- Hansen, James; Sato, Makiko; Hearty, Paul; Ruedy, Reto; et al. (2016). "Ice melt, sea level rise and superstorms: evidence from paleoclimate data, climate modeling, and modern observations that 2 °C global warming could be dangerous". Atmospheric Chemistry and Physics. 16 (6): 3761–3812. arXiv:1602.01393. Bibcode:2016ACP....16.3761H. doi:10.5194/acp-16-3761-2016. ISSN 1680-7316. S2CID 9410444.
{{cite journal}}
: CS1 maint: unflagged free DOI (link) - Harvey, Jeffrey A.; Van den Berg, Daphne; Ellers, Jacintha; Kampen, Remko; et al. (2018). "Internet Blogs, Polar Bears, and Climate-Change Denial by Proxy". BioScience. 68 (4): 281–287. doi:10.1093/biosci/bix133. ISSN 0006-3568. PMC 5894087. PMID 29662248.
- Hawkins, Ed; Ortega, Pablo; Suckling, Emma; Schurer, Andrew; et al. (2017). "Estimating Changes in Global Temperature since the Preindustrial Period". Bulletin of the American Meteorological Society. 98 (9): 1841–1856. Bibcode:2017BAMS...98.1841H. doi:10.1175/bams-d-16-0007.1. ISSN 0003-0007.
- He, Yanyi; Wang, Kaicun; Zhou, Chunlüe; Wild, Martin (2018). "A Revisit of Global Dimming and Brightening Based on the Sunshine Duration". Geophysical Research Letters. 45 (9): 4281–4289. Bibcode:2018GeoRL..45.4281H. doi:10.1029/2018GL077424. ISSN 1944-8007.
- Hilaire, Jérôme; Minx, Jan C.; Callaghan, Max W.; Edmonds, Jae; Luderer, Gunnar; Nemet, Gregory F.; Rogelj, Joeri; Zamora, Maria Mar (17 October 2019). "Negative emissions and international climate goals—learning from and about mitigation scenarios". Climatic Change. 157 (2): 189–219. Bibcode:2019ClCh..157..189H. doi:10.1007/s10584-019-02516-4.
- Hodder, Patrick; Martin, Brian (2009). "Climate Crisis? The Politics of Emergency Framing". Economic and Political Weekly. 44 (36): 53–60. ISSN 0012-9976. JSTOR 25663518.
- Holding, S.; Allen, D. M.; Foster, S.; Hsieh, A.; et al. (2016). "Groundwater vulnerability on small islands". Nature Climate Change. 6 (12): 1100–1103. Bibcode:2016NatCC...6.1100H. doi:10.1038/nclimate3128. ISSN 1758-6798.
- Joo, Gea-Jae; Kim, Ji Yoon; Do, Yuno; Lineman, Maurice (2015). "Talking about Climate Change and Global Warming". PLOS ONE. 10 (9): e0138996. Bibcode:2015PLoSO..1038996L. doi:10.1371/journal.pone.0138996. ISSN 1932-6203. PMC 4587979. PMID 26418127.
- Kabir, Russell; Khan, Hafiz T. A.; Ball, Emma; Caldwell, Khan (2016). "Climate Change Impact: The Experience of the Coastal Areas of Bangladesh Affected by Cyclones Sidr and Aila". Journal of Environmental and Public Health. 2016: 9654753. doi:10.1155/2016/9654753. PMC 5102735. PMID 27867400.
- Kaczan, David J.; Orgill-Meyer, Jennifer (2020). "The impact of climate change on migration: a synthesis of recent empirical insights". Climatic Change. 158 (3): 281–300. Bibcode:2020ClCh..158..281K. doi:10.1007/s10584-019-02560-0. S2CID 207988694. Retrieved 9 February 2021.
- Kennedy, J. J.; Thorne, W. P.; Peterson, T. C.; Ruedy, R. A.; et al. (2010). Arndt, D. S.; Baringer, M. O.; Johnson, M. R. (eds.). "How do we know the world has warmed?". Special supplement: State of the Climate in 2009. Bulletin of the American Meteorological Society. 91 (7). S26-S27. doi:10.1175/BAMS-91-7-StateoftheClimate.
- Kopp, R. E.; Hayhoe, K.; Easterling, D. R.; Hall, T.; et al. (2017). "Chapter 15: Potential Surprises: Compound Extremes and Tipping Elements". In USGCRP 2017. pp. 1–470. Archived from the original on 20 August 2018.
- Kossin, J. P.; Hall, T.; Knutson, T.; Kunkel, K. E.; Trapp, R. J.; Waliser, D. E.; Wehner, M. F. (2017). "Chapter 9: Extreme Storms". In USGCRP2017. pp. 1–470.
- Knutson, T. (2017). "Appendix C: Detection and attribution methodologies overview.". In USGCRP2017. pp. 1–470.
- Kreidenweis, Ulrich; Humpenöder, Florian; Stevanović, Miodrag; Bodirsky, Benjamin Leon; et al. (July 2016). "Afforestation to mitigate climate change: impacts on food prices under consideration of albedo effects". Environmental Research Letters. 11 (8): 085001. Bibcode:2016ERL....11h5001K. doi:10.1088/1748-9326/11/8/085001. ISSN 1748-9326. S2CID 8779827.
- Kvande, H. (2014). "The Aluminum Smelting Process". Journal of Occupational and Environmental Medicine. 56 (5 Suppl): S2–S4. doi:10.1097/JOM.0000000000000154. PMC 4131936. PMID 24806722.
- Lapenis, Andrei G. (1998). "Arrhenius and the Intergovernmental Panel on Climate Change". Eos. 79 (23): 271. Bibcode:1998EOSTr..79..271L. doi:10.1029/98EO00206.
- Levermann, Anders; Clark, Peter U.; Marzeion, Ben; Milne, Glenn A.; et al. (2013). "The multimillennial sea-level commitment of global warming". Proceedings of the National Academy of Sciences. 110 (34): 13745–13750. Bibcode:2013PNAS..11013745L. doi:10.1073/pnas.1219414110. ISSN 0027-8424. PMC 3752235. PMID 23858443.
- Lenoir, Jonathan; Bertrand, Romain; Comte, Lise; Bourgeaud, Luana; et al. (2020). "Species better track climate warming in the oceans than on land". Nature Ecology & Evolution. 4 (8): 1044–1059. doi:10.1038/s41559-020-1198-2. ISSN 2397-334X. PMID 32451428. S2CID 218879068.
- Liepert, Beate G.; Previdi, Michael (2009). "Do Models and Observations Disagree on the Rainfall Response to Global Warming?". Journal of Climate. 22 (11): 3156–3166. Bibcode:2009JCli...22.3156L. doi:10.1175/2008JCLI2472.1.
- Liverman, Diana M. (2009). "Conventions of climate change: constructions of danger and the dispossession of the atmosphere". Journal of Historical Geography. 35 (2): 279–296. doi:10.1016/j.jhg.2008.08.008.
- Loeb, Norman G.; Johnson, Gregory C.; Thorsen, Tyler J.; Lyman, John M.; Rose, Fred G.; Kato, Seiji (2021). "Satellite and Ocean Data Reveal Marked Increase in Earth's Heating Rate". Geophysical Research Letters. 48 (13). American Geophysical Union (AGU). e2021GL093047. Bibcode:2021GeoRL..4893047L. doi:10.1029/2021gl093047. ISSN 0094-8276. S2CID 236233508.
- Mach, Katharine J.; Kraan, Caroline M.; Adger, W. Neil; Buhaug, Halvard; et al. (2019). "Climate as a risk factor for armed conflict". Nature. 571 (7764): 193–197. Bibcode:2019Natur.571..193M. doi:10.1038/s41586-019-1300-6. ISSN 1476-4687. PMID 31189956. S2CID 186207310.
- Matthews, H. Damon; Gillett, Nathan P.; Stott, Peter A.; Zickfeld, Kirsten (2009). "The proportionality of global warming to cumulative carbon emissions". Nature. 459 (7248): 829–832. Bibcode:2009Natur.459..829M. doi:10.1038/nature08047. ISSN 1476-4687. PMID 19516338. S2CID 4423773.
- Matthews, Tom (2018). "Humid heat and climate change". Progress in Physical Geography: Earth and Environment. 42 (3): 391–405. doi:10.1177/0309133318776490. S2CID 134820599.
- McNeill, V. Faye (2017). "Atmospheric Aerosols: Clouds, Chemistry, and Climate". Annual Review of Chemical and Biomolecular Engineering. 8 (1): 427–444. doi:10.1146/annurev-chembioeng-060816-101538. ISSN 1947-5438. PMID 28415861.
- Melillo, J. M.; Frey, S. D.; DeAngelis, K. M.; Werner, W. J.; et al. (2017). "Long-term pattern and magnitude of soil carbon feedback to the climate system in a warming world". Science. 358 (6359): 101–105. Bibcode:2017Sci...358..101M. doi:10.1126/science.aan2874. PMID 28983050.
- Mercure, J.-F.; Pollitt, H.; Viñuales, J. E.; Edwards, N. R.; et al. (2018). "Macroeconomic impact of stranded fossil fuel assets" (PDF). Nature Climate Change. 8 (7): 588–593. Bibcode:2018NatCC...8..588M. doi:10.1038/s41558-018-0182-1. ISSN 1758-6798. S2CID 89799744.
- Mitchum, G. T.; Masters, D.; Hamlington, B. D.; Fasullo, J. T.; et al. (2018). "Climate-change–driven accelerated sea-level rise detected in the altimeter era". Proceedings of the National Academy of Sciences. 115 (9): 2022–2025. Bibcode:2018PNAS..115.2022N. doi:10.1073/pnas.1717312115. ISSN 0027-8424. PMC 5834701. PMID 29440401.
- National Academies of Sciences, Engineering, and Medicine (2019). Negative Emissions Technologies and Reliable Sequestration: A Research Agenda (Report). Washington, D.C.: The National Academies Press. doi:10.17226/25259. ISBN 978-0-309-48455-8.
- National Research Council (2011). "Causes and Consequences of Climate Change". America's Climate Choices. Washington, D.C.: The National Academies Press. doi:10.17226/12781. ISBN 978-0-309-14585-5. Archived from the original on 21 July 2015. Retrieved 28 January 2019.
- Neukom, Raphael; Steiger, Nathan; Gómez-Navarro, Juan José; Wang, Jianghao; et al. (2019a). "No evidence for globally coherent warm and cold periods over the preindustrial Common Era" (PDF). Nature. 571 (7766): 550–554. Bibcode:2019Natur.571..550N. doi:10.1038/s41586-019-1401-2. ISSN 1476-4687. PMID 31341300. S2CID 198494930.
- Neukom, Raphael; Barboza, Luis A.; Erb, Michael P.; Shi, Feng; et al. (2019b). "Consistent multidecadal variability in global temperature reconstructions and simulations over the Common Era". Nature Geoscience. 12 (8): 643–649. Bibcode:2019NatGe..12..643P. doi:10.1038/s41561-019-0400-0. ISSN 1752-0908. PMC 6675609. PMID 31372180.
- O’Neill, Saffron J.; Boykoff, Max (2010). "Climate denier, skeptic, or contrarian?". Proceedings of the National Academy of Sciences of the United States of America. 107 (39): E151. Bibcode:2010PNAS..107E.151O. doi:10.1073/pnas.1010507107. ISSN 0027-8424. PMC 2947866. PMID 20807754.
- Poloczanska, Elvira S.; Brown, Christopher J.; Sydeman, William J.; Kiessling, Wolfgang; et al. (2013). "Global imprint of climate change on marine life" (PDF). Nature Climate Change. 3 (10): 919–925. Bibcode:2013NatCC...3..919P. doi:10.1038/nclimate1958. ISSN 1758-6798.
- Rahmstorf, Stefan; Cazenave, Anny; Church, John A.; Hansen, James E.; et al. (2007). "Recent Climate Observations Compared to Projections" (PDF). Science. 316 (5825): 709. Bibcode:2007Sci...316..709R. doi:10.1126/science.1136843. PMID 17272686. S2CID 34008905. Archived (PDF) from the original on 6 September 2018.
- Ramanathan, V.; Carmichael, G. (2008). "Global and Regional Climate Changes due to Black Carbon". Nature Geoscience. 1 (4): 221–227. Bibcode:2008NatGe...1..221R. doi:10.1038/ngeo156.
- Randel, William J.; Shine, Keith P.; Austin, John; Barnett, John; et al. (2009). "An update of observed stratospheric temperature trends". Journal of Geophysical Research. 114 (D2): D02107. Bibcode:2009JGRD..114.2107R. doi:10.1029/2008JD010421. HAL hal-00355600.
- Rauner, Sebastian; Bauer, Nico; Dirnaichner, Alois; Van Dingenen, Rita; Mutel, Chris; Luderer, Gunnar (2020). "Coal-exit health and environmental damage reductions outweigh economic impacts". Nature Climate Change. 10 (4): 308–312. Bibcode:2020NatCC..10..308R. doi:10.1038/s41558-020-0728-x. ISSN 1758-6798. S2CID 214619069.
- Rogelj, Joeri; Forster, Piers M.; Kriegler, Elmar; Smith, Christopher J.; et al. (2019). "Estimating and tracking the remaining carbon budget for stringent climate targets". Nature. 571 (7765): 335–342. Bibcode:2019Natur.571..335R. doi:10.1038/s41586-019-1368-z. ISSN 1476-4687. PMID 31316194. S2CID 197542084.
- Rogelj, Joeri; Meinshausen, Malte; Schaeffer, Michiel; Knutti, Reto; Riahi, Keywan (2015). "Impact of short-lived non-CO2 mitigation on carbon budgets for stabilizing global warming". Environmental Research Letters. 10 (7): 1–10. Bibcode:2015ERL....10g5001R. doi:10.1088/1748-9326/10/7/075001.
- Ruseva, Tatyana; Hedrick, Jamie; Marland, Gregg; Tovar, Henning; et al. (2020). "Rethinking standards of permanence for terrestrial and coastal carbon: implications for governance and sustainability". Current Opinion in Environmental Sustainability. 45: 69–77. doi:10.1016/j.cosust.2020.09.009. ISSN 1877-3435. S2CID 229069907.
- Samset, B. H.; Sand, M.; Smith, C. J.; Bauer, S. E.; et al. (2018). "Climate Impacts From a Removal of Anthropogenic Aerosol Emissions" (PDF). Geophysical Research Letters. 45 (2): 1020–1029. Bibcode:2018GeoRL..45.1020S. doi:10.1002/2017GL076079. ISSN 1944-8007. PMC 7427631. PMID 32801404.
- Sand, M.; Berntsen, T. K.; von Salzen, K.; Flanner, M. G.; et al. (2015). "Response of Arctic temperature to changes in emissions of short-lived climate forcers". Nature. 6 (3): 286–289. doi:10.1038/nclimate2880.
- Schmidt, Gavin A.; Ruedy, Reto A.; Miller, Ron L.; Lacis, Andy A. (2010). "Attribution of the present-day total greenhouse effect". Journal of Geophysical Research: Atmospheres. 115 (D20): D20106. Bibcode:2010JGRD..11520106S. doi:10.1029/2010JD014287. ISSN 2156-2202. S2CID 28195537.
- Schmidt, Gavin A.; Shindell, Drew T.; Tsigaridis, Kostas (2014). "Reconciling warming trends". Nature Geoscience. 7 (3): 158–160. Bibcode:2014NatGe...7..158S. doi:10.1038/ngeo2105. hdl:2060/20150000726.
- Serdeczny, Olivia; Adams, Sophie; Baarsch, Florent; Coumou, Dim; et al. (2016). "Climate change impacts in Sub-Saharan Africa: from physical changes to their social repercussions" (PDF). Regional Environmental Change. 17 (6): 1585–1600. doi:10.1007/s10113-015-0910-2. ISSN 1436-378X. S2CID 3900505.
- Sutton, Rowan T.; Dong, Buwen; Gregory, Jonathan M. (2007). "Land/sea warming ratio in response to climate change: IPCC AR4 model results and comparison with observations". Geophysical Research Letters. 34 (2): L02701. Bibcode:2007GeoRL..3402701S. doi:10.1029/2006GL028164.
- Smale, Dan A.; Wernberg, Thomas; Oliver, Eric C. J.; Thomsen, Mads; Harvey, Ben P. (2019). "Marine heatwaves threaten global biodiversity and the provision of ecosystem services" (PDF). Nature Climate Change. 9 (4): 306–312. Bibcode:2019NatCC...9..306S. doi:10.1038/s41558-019-0412-1. ISSN 1758-6798. S2CID 91471054.
- Smith, Joel B.; Schneider, Stephen H.; Oppenheimer, Michael; Yohe, Gary W.; et al. (2009). "Assessing dangerous climate change through an update of the Intergovernmental Panel on Climate Change (IPCC) 'reasons for concern'". Proceedings of the National Academy of Sciences. 106 (11): 4133–4137. Bibcode:2009PNAS..106.4133S. doi:10.1073/pnas.0812355106. PMC 2648893. PMID 19251662.
- Smith, N.; Leiserowitz, A. (2013). "The role of emotion in global warming policy support and opposition". Risk Analysis. 34 (5): 937–948. doi:10.1111/risa.12140. PMC 4298023. PMID 24219420.
- Springmann, M.; Mason-D’Croz, D.; Robinson, S.; Garnett, T.; et al. (2016). "Global and regional health effects of future food production under climate change: a modelling study". Lancet. 387 (10031): 1937–1946. doi:10.1016/S0140-6736(15)01156-3. PMID 26947322. S2CID 41851492.
- Stroeve, J.; Holland, Marika M.; Meier, Walt; Scambos, Ted; et al. (2007). "Arctic sea ice decline: Faster than forecast". Geophysical Research Letters. 34 (9): L09501. Bibcode:2007GeoRL..3409501S. doi:10.1029/2007GL029703.
- Storelvmo, T.; Phillips, P. C. B.; Lohmann, U.; Leirvik, T.; Wild, M. (2016). "Disentangling greenhouse warming and aerosol cooling to reveal Earth's climate sensitivity" (PDF). Nature Geoscience. 9 (4): 286–289. Bibcode:2016NatGe...9..286S. doi:10.1038/ngeo2670. ISSN 1752-0908.
- Turetsky, Merritt R.; Abbott, Benjamin W.; Jones, Miriam C.; Anthony, Katey Walter; et al. (2019). "Permafrost collapse is accelerating carbon release". Nature. 569 (7754): 32–34. Bibcode:2019Natur.569...32T. doi:10.1038/d41586-019-01313-4. PMID 31040419.
- Turner, Monica G.; Calder, W. John; Cumming, Graeme S.; Hughes, Terry P.; et al. (2020). "Climate change, ecosystems and abrupt change: science priorities". Philosophical Transactions of the Royal Society B. 375 (1794). doi:10.1098/rstb.2019.0105. PMC 7017767. PMID 31983326.
- Twomey, S. (1977). "The Influence of Pollution on the Shortwave Albedo of Clouds". J. Atmos. Sci. 34 (7): 1149–1152. Bibcode:1977JAtS...34.1149T. doi:10.1175/1520-0469(1977)034<1149:TIOPOT>2.0.CO;2. ISSN 1520-0469.
- Tyndall, John (1861). "On the Absorption and Radiation of Heat by Gases and Vapours, and on the Physical Connection of Radiation, Absorption, and Conduction". Philosophical Magazine. 4. 22: 169–194, 273–285. Archived from the original on 26 March 2016.
- Urban, Mark C. (2015). "Accelerating extinction risk from climate change". Science. 348 (6234): 571–573. Bibcode:2015Sci...348..571U. doi:10.1126/science.aaa4984. ISSN 0036-8075. PMID 25931559.
- USGCRP (2009). Karl, T. R.; Melillo, J.; Peterson, T.; Hassol, S. J. (eds.). Global Climate Change Impacts in the United States. Cambridge University Press. ISBN 978-0-521-14407-0. Archived from the original on 6 April 2010. Retrieved 17 April 2010.
- USGCRP (2017). Wuebbles, D. J.; Fahey, D. W.; Hibbard, K. A.; Dokken, D. J.; et al. (eds.). Climate Science Special Report: Fourth National Climate Assessment, Volume I. Washington, D.C.: U.S. Global Change Research Program. doi:10.7930/J0J964J6.
- Vandyck, T.; Keramidas, K.; Kitous, A.; Spadaro, J.; et al. (2018). "Air quality co-benefits for human health and agriculture counterbalance costs to meet Paris Agreement pledges". Nature Communications. 9 (4939): 4939. Bibcode:2018NatCo...9.4939V. doi:10.1038/s41467-018-06885-9. PMC 6250710. PMID 30467311.
- Wuebbles, D. J.; Easterling, D. R.; Hayhoe, K.; Knutson, T.; et al. (2017). "Chapter 1: Our Globally Changing Climate" (PDF). In USGCRP2017.
- Walsh, John; Wuebbles, Donald; Hayhoe, Katherine; Kossin, Kossin; et al. (2014). "Appendix 3: Climate Science Supplement" (PDF). Climate Change Impacts in the United States: The Third National Climate Assessment.
{{cite book}}
:|work=
ignored (help) - Wang, Bin; Shugart, Herman H.; Lerdau, Manuel T. (2017). "Sensitivity of global greenhouse gas budgets to tropospheric ozone pollution mediated by the biosphere". Environmental Research Letters. 12 (8): 084001. Bibcode:2017ERL....12h4001W. doi:10.1088/1748-9326/aa7885. ISSN 1748-9326.
- Watts, Nick; Adger, W Neil; Agnolucci, Paolo; Blackstock, Jason; et al. (2015). "Health and climate change: policy responses to protect public health". The Lancet. 386 (10006): 1861–1914. doi:10.1016/S0140-6736(15)60854-6. hdl:10871/20783. PMID 26111439. S2CID 205979317. Archived from the original on 7 April 2017.
- Watts, Nick; Amann, Markus; Arnell, Nigel; Ayeb-Karlsson, Sonja; et al. (2019). "The 2019 report of The Lancet Countdown on health and climate change: ensuring that the health of a child born today is not defined by a changing climate". The Lancet. 394 (10211): 1836–1878. doi:10.1016/S0140-6736(19)32596-6. ISSN 0140-6736. PMID 31733928. S2CID 207976337.
- Weart, Spencer (2013). "Rise of interdisciplinary research on climate". Proceedings of the National Academy of Sciences. 110 (Supplement 1): 3657–3664. doi:10.1073/pnas.1107482109. PMC 3586608. PMID 22778431.
- Wild, M.; Gilgen, Hans; Roesch, Andreas; Ohmura, Atsumu; et al. (2005). "From Dimming to Brightening: Decadal Changes in Solar Radiation at Earth's Surface". Science. 308 (5723): 847–850. Bibcode:2005Sci...308..847W. doi:10.1126/science.1103215. PMID 15879214. S2CID 13124021.
- Williams, Richard G; Ceppi, Paulo; Katavouta, Anna (2020). "Controls of the transient climate response to emissions by physical feedbacks, heat uptake and carbon cycling". Environmental Research Letters. 15 (9): 0940c1. Bibcode:2020ERL....15i40c1W. doi:10.1088/1748-9326/ab97c9.
- Wolff, Eric W.; Shepherd, John G.; Shuckburgh, Emily; Watson, Andrew J. (2015). "Feedbacks on climate in the Earth system: introduction". Philosophical Transactions of the Royal Society A: Mathematical, Physical and Engineering Sciences. 373 (2054): 20140428. Bibcode:2015RSPTA.37340428W. doi:10.1098/rsta.2014.0428. PMC 4608041. PMID 26438277.
- Zeng, Ning; Yoon, Jinho (2009). "Expansion of the world's deserts due to vegetation-albedo feedback under global warming". Geophysical Research Letters. 36 (17): L17401. Bibcode:2009GeoRL..3617401Z. doi:10.1029/2009GL039699. ISSN 1944-8007. S2CID 1708267.
- Zhang, Jinlun; Lindsay, Ron; Steele, Mike; Schweiger, Axel (2008). "What drove the dramatic arctic sea ice retreat during summer 2007?". Geophysical Research Letters. 35 (11): 1–5. Bibcode:2008GeoRL..3511505Z. doi:10.1029/2008gl034005. S2CID 9387303.
- Zhao, C.; Liu, B.; et al. (2017). "Temperature increase reduces global yields of major crops in four independent estimates". Proceedings of the National Academy of Sciences. 114 (35): 9326–9331. Bibcode:2017PNAS..114.9326Z. doi:10.1073/pnas.1701762114. PMC 5584412. PMID 28811375.
Books, reports and legal documents
- Academia Brasileira de Ciéncias (Brazil); Royal Society of Canada; Chinese Academy of Sciences; Académie des Sciences (France); Deutsche Akademie der Naturforscher Leopoldina (Germany); Indian National Science Academy; Accademia Nazionale dei Lincei (Italy); Science Council of Japan, Academia Mexicana de Ciencias; Academia Mexicana de Ciencias (Mexico); Russian Academy of Sciences; Academy of Science of South Africa; Royal Society (United Kingdom); National Academy of Sciences (United States of America) (May 2009). "G8+5 Academies' joint statement: Climate change and the transformation of energy technologies for a low carbon future" (PDF). The National Academies of Sciences, Engineering, and Medicine. Archived from the original (PDF) on 15 February 2010. Retrieved 5 May 2010.
- Archer, David; Pierrehumbert, Raymond (2013). The Warming Papers: The Scientific Foundation for the Climate Change Forecast. John Wiley & Sons. ISBN 978-1-118-68733-8.
- Bridle, Richard; Sharma, Shruti; Mostafa, Mostafa; Geddes, Anna (June 2019). Fossil Fuel to Clean Energy Subsidy Swaps (PDF) (Report).
- Climate Focus (December 2015). "The Paris Agreement: Summary. Climate Focus Client Brief on the Paris Agreement III" (PDF). Archived (PDF) from the original on 5 October 2018. Retrieved 12 April 2019.
- Clark, P. U.; Weaver, A. J.; Brook, E.; Cook, E. R.; et al. (December 2008). "Executive Summary". In: Abrupt Climate Change. A Report by the U.S. Climate Change Science Program and the Subcommittee on Global Change Research. Reston, VA: U.S. Geological Survey. Archived from the original on 4 May 2013.
- Conceição; et al. (2020). Human Development Report 2020 The Next Frontier: Human Development and the Anthropocene (PDF) (Report). United Nations Development Programme. Retrieved 9 January 2021.
- DeFries, Ruth; Edenhofer, Ottmar; Halliday, Alex; Heal, Geoffrey; et al. (September 2019). The missing economic risks in assessments of climate change impacts (PDF) (Report). Grantham Research Institute on Climate Change and the Environment, London School of Economics and Political Science.
- Dessler, Andrew E. and Edward A. Parson, eds. The science and politics of global climate change: A guide to the debate (Cambridge University Press, 2019).
- Dessai, Suraje (2001). "The climate regime from The Hague to Marrakech: Saving or sinking the Kyoto Protocol?" (PDF). Tyndall Centre Working Paper 12. Tyndall Centre. Archived from the original (PDF) on 10 June 2012. Retrieved 5 May 2010.
- Dunlap, Riley E.; McCright, Aaron M. (2011). "Chapter 10: Organized climate change denial". In Dryzek, John S.; Norgaard, Richard B.; Schlosberg, David (eds.). The Oxford Handbook of Climate Change and Society. Oxford University Press. pp. 144–160. ISBN 978-0-19-956660-0.
- Dunlap, Riley E.; McCright, Aaron M. (2015). "Chapter 10: Challenging Climate Change: The Denial Countermovement". In Dunlap, Riley E.; Brulle, Robert J. (eds.). Climate Change and Society: Sociological Perspectives. Oxford University Press. pp. 300–332. ISBN 978-0199356119.
- European Commission (28 November 2018). In-depth analysis accompanying the Commission Communication COM(2018) 773: A Clean Planet for all – A European strategic long-term vision for a prosperous, modern, competitive and climate neutral economy (PDF) (Report). Brussels. p. 188.
- Flavell, Alex (2014). IOM outlook on migration, environment and climate change (PDF) (Report). Geneva, Switzerland: International Organization for Migration (IOM). ISBN 978-92-9068-703-0. OCLC 913058074.
- Fleming, James Rodger (2007). The Callendar Effect: the life and work of Guy Stewart Callendar (1898–1964). Boston: American Meteorological Society. ISBN 978-1-878220-76-9.
- Flynn, C.; Yamasumi, E.; Fisher, S.; Snow, D.; et al. (January 2021). Peoples' Climate Vote (PDF) (Report). UNDP and University of Oxford. Retrieved 5 August 2021.
- Global Methane Initiative (2020). Global Methane Emissions and Mitigation Opportunities (PDF) (Report). Global Methane Initiative.
- Hallegatte, Stephane; Bangalore, Mook; Bonzanigo, Laura; Fay, Marianne; et al. (2016). Shock Waves : Managing the Impacts of Climate Change on Poverty. Climate Change and Development (PDF). Washington, D.C.: World Bank. doi:10.1596/978-1-4648-0673-5. hdl:10986/22787. ISBN 978-1-4648-0674-2.
- Haywood, Jim (2016). "Chapter 27 – Atmospheric Aerosols and Their Role in Climate Change". In Letcher, Trevor M. (ed.). Climate Change: Observed Impacts on Planet Earth. Elsevier. ISBN 978-0-444-63524-2.
- IEA (December 2020). "COVID-19 and energy efficiency". Energy Efficiency 2020 (Report). Paris, France. Retrieved 6 April 2021.
- IEA (October 2021). Net Zero By 2050: A Roadmap for the Global Energy Sector (PDF) (Report). Paris, France. Retrieved 4 April 2022.
- Krogstrup, Signe; Oman, William (4 September 2019). Macroeconomic and Financial Policies for Climate Change Mitigation: A Review of the Literature (PDF). IMF working papers. doi:10.5089/9781513511955.001. ISBN 978-1-5135-1195-5. ISSN 1018-5941. S2CID 203245445.
- Leiserowitz, A.; Carman, J.; Buttermore, N.; Wang, X.; et al. (2021). International Public Opinion on Climate Change (PDF) (Report). New Haven, CT: Yale Program on Climate Change Communication and Facebook Data for Good. Retrieved 5 August 2021.
- Letcher, Trevor M., ed. (2020). Future Energy: Improved, Sustainable and Clean Options for our Planet (Third ed.). Elsevier. ISBN 978-0-08-102886-5.
- Meinshausen, Malte (2019). "Implications of the Developed Scenarios for Climate Change". In Teske, Sven (ed.). Achieving the Paris Climate Agreement Goals: Global and Regional 100% Renewable Energy Scenarios with Non-energy GHG Pathways for +1.5 °C and +2 °C. Springer International Publishing. pp. 459–469. doi:10.1007/978-3-030-05843-2_12. ISBN 978-3-030-05843-2. S2CID 133868222.
- Miller, J.; Du, L.; Kodjak, D. (2017). Impacts of World-Class Vehicle Efficiency and Emissions Regulations in Select G20 Countries (PDF) (Report). Washington, D.C.: The International Council on Clean Transportation.
- Müller, Benito (February 2010). Copenhagen 2009: Failure or final wake-up call for our leaders? EV 49 (PDF). Oxford Institute for Energy Studies. p. i. ISBN 978-1-907555-04-6. Archived (PDF) from the original on 10 July 2017. Retrieved 18 May 2010.
- National Academies (2008). Understanding and responding to climate change: Highlights of National Academies Reports, 2008 edition (PDF) (Report). National Academy of Sciences. Archived from the original (PDF) on 11 October 2017. Retrieved 9 November 2010.
- National Research Council (2012). Climate Change: Evidence, Impacts, and Choices (PDF) (Report). Washington, D.C.: National Academy of Sciences. Archived from the original (PDF) on 20 February 2013. Retrieved 9 September 2017.
- Newell, Peter (14 December 2006). Climate for Change: Non-State Actors and the Global Politics of the Greenhouse. Cambridge University Press. ISBN 978-0-521-02123-4. Retrieved 30 July 2018.
- NOAA. "January 2017 analysis from NOAA: Global and Regional Sea Level Rise Scenarios for the United States" (PDF). Archived (PDF) from the original on 18 December 2017. Retrieved 7 February 2019.
- Olivier, J. G. J.; Peters, J. A. H. W. (2019). Trends in global CO2 and total greenhouse gas emissions (PDF). The Hague: PBL Netherlands Environmental Assessment Agency.
- Oreskes, Naomi (2007). "The scientific consensus on climate change: How do we know we're not wrong?". In DiMento, Joseph F. C.; Doughman, Pamela M. (eds.). Climate Change: What It Means for Us, Our Children, and Our Grandchildren. The MIT Press. ISBN 978-0-262-54193-0.
- Oreskes, Naomi; Conway, Erik (2010). Merchants of Doubt: How a Handful of Scientists Obscured the Truth on Issues from Tobacco Smoke to Global Warming (first ed.). Bloomsbury Press. ISBN 978-1-59691-610-4.
- Pew Research Center (November 2015). Global Concern about Climate Change, Broad Support for Limiting Emissions (PDF) (Report). Retrieved 5 August 2021.
- REN21 (2020). Renewables 2020 Global Status Report (PDF). Paris: REN21 Secretariat. ISBN 978-3-948393-00-7.
{{cite book}}
: CS1 maint: numeric names: authors list (link) - Royal Society (13 April 2005). Economic Affairs – Written Evidence. The Economics of Climate Change, the Second Report of the 2005–2006 session, produced by the UK Parliament House of Lords Economics Affairs Select Committee. UK Parliament. Archived from the original on 13 November 2011. Retrieved 9 July 2011.
- Setzer, Joana; Byrnes, Rebecca (July 2019). Global trends in climate change litigation: 2019 snapshot (PDF). London: the Grantham Research Institute on Climate Change and the Environment and the Centre for Climate Change Economics and Policy.
- Steinberg, D.; Bielen, D.; et al. (July 2017). Electrification & Decarbonization: Exploring U.S. Energy Use and Greenhouse Gas Emissions in Scenarios with Widespread Electrification and Power Sector Decarbonization (PDF) (Report). Golden, Colorado: National Renewable Energy Laboratory.
- Teske, Sven, ed. (2019). "Executive Summary" (PDF). Achieving the Paris Climate Agreement Goals: Global and Regional 100% Renewable Energy Scenarios with Non-energy GHG Pathways for +1.5 °C and +2 °C. Springer International Publishing. pp. xiii–xxxv. doi:10.1007/978-3-030-05843-2. ISBN 978-3-030-05843-2. S2CID 198078901.
- Teske, Sven; Pregger, Thomas; Naegler, Tobias; Simon, Sonja; et al. (2019). "Energy Scenario Results". In Teske, Sven (ed.). Achieving the Paris Climate Agreement Goals: Global and Regional 100% Renewable Energy Scenarios with Non-energy GHG Pathways for +1.5 °C and +2 °C. Springer International Publishing. pp. 175–402. doi:10.1007/978-3-030-05843-2_8. ISBN 978-3-030-05843-2.
- Teske, Sven (2019). "Trajectories for a Just Transition of the Fossil Fuel Industry". In Teske, Sven (ed.). Achieving the Paris Climate Agreement Goals: Global and Regional 100% Renewable Energy Scenarios with Non-energy GHG Pathways for +1.5 °C and +2 °C. Springer International Publishing. pp. 403–411. doi:10.1007/978-3-030-05843-2_9. ISBN 978-3-030-05843-2. S2CID 133961910.
- UN FAO (2016). Global Forest Resources Assessment 2015. How are the world's forests changing? (PDF) (Report). Food and Agriculture Organization of the United Nations. ISBN 978-92-5-109283-5. Retrieved 1 December 2019.
- United Nations Environment Programme (2019). Emissions Gap Report 2019 (PDF). Nairobi. ISBN 978-92-807-3766-0.
{{cite book}}
: CS1 maint: location missing publisher (link) - United Nations Environment Programme (2021). Emissions Gap Report 2021 (PDF). Nairobi. ISBN 978-92-807-3890-2.
{{cite book}}
: CS1 maint: location missing publisher (link) - UNEP (2018). The Adaptation Gap Report 2018. Nairobi, Kenya: United Nations Environment Programme (UNEP). ISBN 978-92-807-3728-8.
- UNFCCC (1992). United Nations Framework Convention on Climate Change (PDF).
- UNFCCC (1997). "Kyoto Protocol to the United Nations Framework Convention on Climate Change". United Nations.
- UNFCCC (30 March 2010). "Decision 2/CP.15: Copenhagen Accord". Report of the Conference of the Parties on its fifteenth session, held in Copenhagen from 7 to 19 December 2009. United Nations Framework Convention on Climate Change. FCCC/CP/2009/11/Add.1. Archived from the original on 30 April 2010. Retrieved 17 May 2010.
- UNFCCC (2015). "Paris Agreement" (PDF). United Nations Framework Convention on Climate Change.
- UNFCCC (26 February 2021). Nationally determined contributions under the Paris Agreement Synthesis report by the secretariat (PDF) (Report). United Nations Framework Convention on Climate Change.
- Park, Susin (May 2011). "Climate Change and the Risk of Statelessness: The Situation of Low-lying Island States" (PDF). United Nations High Commissioner for Refugees. Archived (PDF) from the original on 2 May 2013. Retrieved 13 April 2012.
- United States Environmental Protection Agency (2016). Methane and Black Carbon Impacts on the Arctic: Communicating the Science (Report). Archived from the original on 6 September 2017. Retrieved 27 February 2019.
- Van Oldenborgh, Geert-Jan; Philip, Sjoukje; Kew, Sarah; Vautard, Robert; et al. (2019). "Human contribution to the record-breaking June 2019 heat wave in France". Semantic Scholar. S2CID 199454488.
- Weart, Spencer (October 2008). The Discovery of Global Warming (2nd ed.). Cambridge, MA: Harvard University Press. ISBN 978-0-674-03189-0. Archived from the original on 18 November 2016. Retrieved 16 June 2020.
- Weart, Spencer (February 2019). The Discovery of Global Warming (online ed.). Archived from the original on 18 June 2020. Retrieved 19 June 2020.
- Weart, Spencer (January 2020). "The Carbon Dioxide Greenhouse Effect". The Discovery of Global Warming. American Institute of Physics. Archived from the original on 11 November 2016. Retrieved 19 June 2020.
- Weart, Spencer (January 2020). "The Public and Climate Change". The Discovery of Global Warming. American Institute of Physics. Archived from the original on 11 November 2016. Retrieved 19 June 2020.
- Weart, Spencer (January 2020). "The Public and Climate Change: Suspicions of a Human-Caused Greenhouse (1956–1969)". The Discovery of Global Warming. American Institute of Physics. Archived from the original on 11 November 2016. Retrieved 19 June 2020.
- Weart, Spencer (January 2020). "The Public and Climate Change (cont. – since 1980)". The Discovery of Global warming. American Institute of Physics. Archived from the original on 11 November 2016. Retrieved 19 June 2020.
- Weart, Spencer (January 2020). "The Public and Climate Change: The Summer of 1988". The Discovery of Global Warming. American Institute of Physics. Archived from the original on 11 November 2016. Retrieved 19 June 2020.
- State and Trends of Carbon Pricing 2019 (PDF) (Report). Washington, D.C.: World Bank. June 2019. doi:10.1596/978-1-4648-1435-8. hdl:10986/29687. ISBN 978-1-4648-1435-8.
- World Health Organization (2014). Quantitative risk assessment of the effects of climate change on selected causes of death, 2030s and 2050s (PDF) (Report). Geneva, Switzerland. ISBN 978-92-4-150769-1.
- World Health Organization (2016). Ambient air pollution: a global assessment of exposure and burden of disease (Report). Geneva, Switzerland. ISBN 978-92-4-1511353.
- World Health Organization (2018). COP24 Special Report Health and Climate Change (PDF). Geneva. ISBN 978-92-4-151497-2.
{{cite book}}
: CS1 maint: location missing publisher (link) - World Meteorological Organization (2021). WMO Statement on the State of the Global Climate in 2020. WMO-No. 1264. Geneva. ISBN 978-92-63-11264-4.
{{cite book}}
: CS1 maint: location missing publisher (link) - World Resources Institute (December 2019). Creating a Sustainable Food Future: A Menu of Solutions to Feed Nearly 10 Billion People by 2050 (PDF). Washington, D.C. ISBN 978-1-56973-953-2.
{{cite book}}
: CS1 maint: location missing publisher (link)
Non-technical sources
- Associated Press
- Colford, Paul (22 September 2015). "An addition to AP Stylebook entry on global warming". AP Style Blog. Retrieved 6 November 2019.
- BBC
- "UK Parliament declares climate change emergency". BBC. 1 May 2019. Retrieved 30 June 2019.
- Rigby, Sara (3 February 2020). "Climate change: should we change the terminology?". BBC Science Focus Magazine. Retrieved 24 March 2020.
- Bulletin of the Atomic Scientists
- Stover, Dawn (23 September 2014). "The global warming 'hiatus'". Bulletin of the Atomic Scientists. Archived from the original on 11 July 2020.
- Carbon Brief
- Yeo, Sophie (4 January 2017). "Clean energy: The challenge of achieving a 'just transition' for workers". Carbon Brief. Retrieved 18 May 2020.
- McSweeney, Robert M.; Hausfather, Zeke (15 January 2018). "Q&A: How do climate models work?". Carbon Brief. Archived from the original on 5 March 2019. Retrieved 2 March 2019.
- Hausfather, Zeke (19 April 2018). "Explainer: How 'Shared Socioeconomic Pathways' explore future climate change". Carbon Brief. Retrieved 20 July 2019.
- Hausfather, Zeke (8 October 2018). "Analysis: Why the IPCC 1.5C report expanded the carbon budget". Carbon Brief. Retrieved 28 July 2020.
- Dunne, Daisy; Gabbatiss, Josh; Mcsweeny, Robert (7 January 2020). "Media reaction: Australia's bushfires and climate change". Carbon Brief. Retrieved 11 January 2020.
- Deutsche Welle
- Ruiz, Irene Banos (22 June 2019). "Climate Action: Can We Change the Climate From the Grassroots Up?". Ecowatch. Deutsche Welle. Archived from the original on 23 June 2019. Retrieved 23 June 2019.
- EPA
- "Myths vs. Facts: Denial of Petitions for Reconsideration of the Endangerment and Cause or Contribute Findings for Greenhouse Gases under Section 202(a) of the Clean Air Act". U.S. Environmental Protection Agency. 25 August 2016. Retrieved 7 August 2017.
- US EPA (13 September 2019). "Global Greenhouse Gas Emissions Data". Archived from the original on 18 February 2020. Retrieved 8 August 2020.
- US EPA (15 September 2020). "Overview of Greenhouse Gases". Retrieved 15 September 2020.
- EUobserver
- "Copenhagen failure 'disappointing', 'shameful'". euobserver.com. 20 December 2009. Archived from the original on 12 April 2019. Retrieved 12 April 2019.
- European Parliament
- Ciucci, M. (February 2020). "Renewable Energy". European Parliament. Retrieved 3 June 2020.
- The Guardian
- Nuccitelli, Dana (26 January 2015). "Climate change could impact the poor much more than previously thought". The Guardian. Archived from the original on 28 December 2016.
- Carrington, Damian (19 March 2019). "School climate strikes: 1.4 million people took part, say campaigners". The Guardian. Archived from the original on 20 March 2019. Retrieved 12 April 2019.
- Rankin, Jennifer (28 November 2019). "'Our house is on fire': EU parliament declares climate emergency". The Guardian. ISSN 0261-3077. Retrieved 28 November 2019.
- Watts, Jonathan (19 February 2020). "Oil and gas firms 'have had far worse climate impact than thought'". The Guardian.
- Carrington, Damian (6 April 2020). "New renewable energy capacity hit record levels in 2019". The Guardian. Retrieved 25 May 2020.
- McCurry, Justin (28 October 2020). "South Korea vows to go carbon neutral by 2050 to fight climate emergency". The Guardian. Retrieved 6 December 2020.
- International Energy Agency
- "Projected Costs of Generating Electricity 2020". IEA. Retrieved 4 April 2022.
- NASA
- "Arctic amplification". NASA. 2013. Archived from the original on 31 July 2018.
- Carlowicz, Michael (12 September 2018). "Watery heatwave cooks the Gulf of Maine". NASA's Earth Observatory.
- Conway, Erik M. (5 December 2008). "What's in a Name? Global Warming vs. Climate Change". NASA. Archived from the original on 9 August 2010.
- Riebeek, H. (16 June 2011). "The Carbon Cycle: Feature Articles: Effects of Changing the Carbon Cycle". Earth Observatory, part of the EOS Project Science Office located at NASA Goddard Space Flight Center. Archived from the original on 6 February 2013. Retrieved 4 February 2013.
- Shaftel, Holly (January 2016). "What's in a name? Weather, global warming and climate change". NASA Climate Change: Vital Signs of the Planet. Archived from the original on 28 September 2018. Retrieved 12 October 2018.
- Shaftel, Holly; Jackson, Randal; Callery, Susan; Bailey, Daniel, eds. (7 July 2020). "Overview: Weather, Global Warming and Climate Change". Climate Change: Vital Signs of the Planet. Retrieved 14 July 2020.
- National Conference of State Legislators
- "State Renewable Portfolio Standards and Goals". National Conference of State Legislators. 17 April 2020. Retrieved 3 June 2020.
- National Geographic
- Welch, Craig (13 August 2019). "Arctic permafrost is thawing fast. That affects us all". National Geographic. Retrieved 25 August 2019.
- National Science Digital Library
- Fleming, James R. (17 March 2008). "Climate Change and Anthropogenic Greenhouse Warming: A Selection of Key Articles, 1824–1995, with Interpretive Essays". National Science Digital Library Project Archive PALE:ClassicArticles. Retrieved 7 October 2019.
- Natural Resources Defense Council
- "What Is the Clean Power Plan?". Natural Resources Defense Council. 29 September 2017. Retrieved 3 August 2020.
- Nature
- Crucifix, Michel (2016). "Earth's narrow escape from a big freeze". Nature. 529 (7585): 162–163. doi:10.1038/529162a. ISSN 1476-4687. PMID 26762453.
- The New York Times
- Rudd, Kevin (25 May 2015). "Paris Can't Be Another Copenhagen". The New York Times. Archived from the original on 3 February 2018. Retrieved 26 May 2015.
- NOAA
- NOAA (10 July 2011). "Polar Opposites: the Arctic and Antarctic". Archived from the original on 22 February 2019. Retrieved 20 February 2019.
- Huddleston, Amara (17 July 2019). "Happy 200th birthday to Eunice Foote, hidden climate science pioneer". NOAA Climate.gov. Retrieved 8 October 2019.
- Our World in Data
- Ritchie, Hannah; Roser, Max (15 January 2018). "Land Use". Our World in Data. Retrieved 1 December 2019.
- Ritchie, Hannah (18 September 2020). "Sector by sector: where do global greenhouse gas emissions come from?". Our World in Data. Retrieved 28 October 2020.
- Roser, Max (2022). "Why did renewables become so cheap so fast?". Our World in Data. Retrieved 4 April 2022.
- Pew Research Center
- Pew Research Center (16 October 2020). "Many globally are as concerned about climate change as about the spread of infectious diseases". Retrieved 19 August 2021.
- Politico
- Tamma, Paola; Schaart, Eline; Gurzu, Anca (11 December 2019). "Europe's Green Deal plan unveiled". Politico. Retrieved 29 December 2019.
- RIVM
- Documentary Sea Blind (Dutch Television) (in Dutch). RIVM: Netherlands National Institute for Public Health and the Environment. 11 October 2016. Archived from the original on 17 August 2018. Retrieved 26 February 2019.
- Salon
- Leopold, Evelyn (25 September 2019). "How leaders planned to avert climate catastrophe at the UN (while Trump hung out in the basement)". Salon. Retrieved 20 November 2019.
- ScienceBlogs
- Gleick, Peter (7 January 2017). "Statements on Climate Change from Major Scientific Academies, Societies, and Associations (January 2017 update)". ScienceBlogs. Retrieved 2 April 2020.
- Scientific American
- Ogburn, Stephanie Paige (29 April 2014). "Indian Monsoons Are Becoming More Extreme". Scientific American. Archived from the original on 22 June 2018.
- Smithsonian
- Wing, Scott L. (29 June 2016). "Studying the Climate of the Past Is Essential for Preparing for Today's Rapidly Changing Climate". Smithsonian. Retrieved 8 November 2019.
- The Sustainability Consortium
- "One-Fourth of Global Forest Loss Permanent: Deforestation Is Not Slowing Down". The Sustainability Consortium. 13 September 2018. Retrieved 1 December 2019.
- UN Environment
- "Curbing environmentally unsafe, irregular and disorderly migration". UN Environment. 25 October 2018. Archived from the original on 18 April 2019. Retrieved 18 April 2019.
- UNFCCC
- "What are United Nations Climate Change Conferences?". UNFCCC. Archived from the original on 12 May 2019. Retrieved 12 May 2019.
- "What is the United Nations Framework Convention on Climate Change?". UNFCCC.
- Union of Concerned Scientists
- "Carbon Pricing 101". Union of Concerned Scientists. 8 January 2017. Retrieved 15 May 2020.
- Vice
- Segalov, Michael (2 May 2019). "The UK Has Declared a Climate Emergency: What Now?". Vice. Retrieved 30 June 2019.
- The Verge
- Calma, Justine (27 December 2019). "2019 was the year of 'climate emergency' declarations". The Verge. Retrieved 28 March 2020.
- Vox
- Roberts, D. (20 September 2019). "Getting to 100% renewables requires cheap energy storage. But how cheap?". Vox. Retrieved 28 May 2020.
- World Health Organization
- "WHO calls for urgent action to protect health from climate change – Sign the call". World Health Organization. November 2015. Archived from the original on 3 January 2021. Retrieved 2 September 2020.
- World Resources Institute
- Butler, Rhett A. (31 March 2021). "Global forest loss increases in 2020". Mongabay. Archived from the original on 1 April 2021. ● Mongabay graphing WRI data from "Forest Loss / How much tree cover is lost globally each year?". research.WRI.org. World Resources Institute — Global Forest Review. January 2021. Archived from the original on 10 March 2021.
- Levin, Kelly (8 August 2019). "How Effective Is Land At Removing Carbon Pollution? The IPCC Weighs In". World Resources institute. Retrieved 15 May 2020.
- Seymour, Frances; Gibbs, David (8 December 2019). "Forests in the IPCC Special Report on Land Use: 7 Things to Know". World Resources Institute.
- Yale Climate Connections
- Peach, Sara (2 November 2010). "Yale Researcher Anthony Leiserowitz on Studying, Communicating with American Public". Yale Climate Connections. Archived from the original on 7 February 2019. Retrieved 30 July 2018.
External links
Listen to this article (1 hour and 16 minutes)

Global warming
- Met Office: Climate Guide – UK National Weather Service
- Global Climate Change Indicators – NOAA
- Up-to-the-second assessment of human-induced global warming since the second half of the 19th century – Oxford University
- Global warming, britannica.com
Definitions from Wiktionary
Media from Commons
News from Wikinews
Quotations from Wikiquote
Texts from Wikisource
Textbooks from Wikibooks
Resources from Wikiversity
Human impact on the environment | |
---|---|
General | |
Causes |
|
Effects |
|
Mitigation |
|
Earth | |
---|---|
Atmosphere | |
Climate | |
Continents | |
Culture and society | |
Environment | |
Geodesy | |
Geophysics | |
Geology | |
Oceans | |
Planetary science | |
Global catastrophic risks | |||||
---|---|---|---|---|---|
Technological | |||||
Sociological | |||||
Ecological |
| ||||
Biological |
| ||||
Astronomical | |||||
Eschatological | |||||
Others |
| ||||
Fictional | |||||
Organizations | |||||
General | |||||
Population | |
---|---|
Major topics | |
Population biology | |
Population ecology | |
Society and population | |
Publications | |
Lists | |
Events and organizations |
|
Related topics | |