
Fused filament fabrication (FFF), also known as fused deposition modeling (with the trademarked acronym FDM), or filament freeform fabrication, is a 3D printing process that uses a continuous filament of a thermoplastic material. Filament is fed from a large spool through a moving, heated printer extruder head, and is deposited on the growing work. The print head is moved under computer control to define the printed shape. Usually the head moves in two dimensions to deposit one horizontal plane, or layer, at a time; the work or the print head is then moved vertically by a small amount to begin a new layer. The speed of the extruder head may also be controlled to stop and start deposition and form an interrupted plane without stringing or dribbling between sections. "Fused filament fabrication" was coined by the members of the RepRap project to give an acronym (FFF) that would be legally unconstrained in its use.
Fused filament printing is now the most popular process (by number of machines) for hobbyist-grade 3D printing. Other techniques such as photopolymerisation and powder sintering may offer better results, but they are much more costly.

The 3D printer head or 3D printer extruder is a part in material extrusion additive manufacturing responsible for raw material melting or softening and forming it into a continuous profile. A wide variety of filament materials are extruded, including thermoplastics such as acrylonitrile butadiene styrene (ABS), polylactic acid (PLA), polyethylene terephthalate glycol (PETG), polyethylene terephthalate (PET), high-impact polystyrene (HIPS), thermoplastic polyurethane (TPU) and aliphatic polyamides (nylon).
History

Fused deposition modeling was developed by S. Scott Crump, co-founder of Stratasys, in 1988. With the 2009 expiration of the patent on this technology, people could use this type of printing without paying Stratasys for the right to do so, opening up commercial, DIY, and open-source (RepRap) 3D printer applications. This has led to a two-orders-of-magnitude price drop since this technology's creation. Stratasys still owns the trademark on the term "FDM".
Process
![]() | This section is missing information about post-processing (solvent smoothing, annealing/remelting, etc.). Please expand the section to include this information. Further details may exist on the talk page. (December 2020) |
3D printing, also referred to as additive manufacturing (AM), involves manufacturing a part by depositing material layer by layer. There is a wide array of different AM technologies that can do this, including material extrusion, binder jetting, material jetting and directed energy deposition. These processes have varied types of extruders and extrude different materials to achieve the final product.
Material extrusion


Fused filament fabrication uses material extrusion to print items, where a feedstock material is pushed through an extruder. In most fused filament fabrication 3D printing machines, the feedstock material comes in the form of a filament wound onto a spool.
The 3D printer liquefier is the component predominantly used in this type of printing. Extruders for these printers have a cold end and a hot end. The cold end pulls material from the spool, using gear- or roller-based torque to the material and controlling the feed rate by means of a stepper motor. The cold end pushes feedstock into the hot end. The hot end consists of a heating chamber and a nozzle. The heating chamber hosts the liquefier, which melts the feedstock to transform it into a liquid. It allows the molten material to exit from the small nozzle to form a thin, tacky bead of plastic that will adhere to the material it is laid on. The nozzle will usually have a diameter of between 0.3 mm and 1.0 mm. Different types of nozzles and heating methods are used depending upon the material to be printed.
Different types of nozzles have different ways of replacing them. The most common used nozzles are the V6 nozzles made popular by E3D and MK8 nozzles. Changing the nozzle must be done while hot, to avoid plastic leaks.
Variants of the process
- Hot extrusion of rods - In these types of 3d printing machines, the feedstock is in form of a rod instead of a filament. Since the rod is thicker than the filament, it can be pushed towards the hot end by means of a piston or rollers, applying a greater force and/or velocity compared to conventional FFF.
- Cold extrusion of slurries - In these types of 3D printing machines, the feedstock comes in form of a slurry, a paste or a clay—all of which are viscous suspension of solid powder particles in a liquid medium, which is dried after deposition. In this case, the material is generally pushed towards the nozzle by the action of a piston, and the nozzle is not heated. Paste-like materials such as ceramics and chocolate can be extruded using the fused filament process and a specialized paste extruder.
- Hot extrusion of pellets - In these types of 3d printing machines the feedstock comes in form of pellets, i.e. small granules of thermoplastic material or mixtures of thermoplastic binder with powder fillers. The material is pushed towards the nozzle by the action of a piston or a rotating screw, which are contained by an extrusion barrel. In this case the whole extrusion barrel is heated, along with the nozzle.
Printing


FFF begins with a software process which processes an STL file, orienting the model for the build process and mathematically slicing the model according to the processing parameters selected. If required, support structures may be generated.
The nozzle can be moved in both horizontal and vertical directions, and is mounted to a mechanical stage, which can be moved in the xy plane.

As the nozzle is moved over the table in a prescribed geometry, it deposits a thin bead of extruded plastic, called a ‘‘road’’ which solidifies quickly upon contact with the substrate and/or roads deposited earlier. Solid layers are generated by following a rasterizing motion where the roads are deposited side by side within an enveloping domain boundary.
Stepper motors or servo motors are typically employed to move the extrusion head. The mechanism used is often an X-Y-Z rectilinear design, although other mechanical designs such as deltabot have been employed.
Once a layer is completed, the platform is lowered in the z direction in order to start the next layer. This process continues until the fabrication of the object is completed.
For successful bonding of the roads in the process, thermal control of the deposited material is necessary. The system can be kept inside a chamber, maintained at a temperature below the melting point of the material being deposited.
Although as a printing technology FFF is very flexible, and it is capable of dealing with small overhangs by the support from lower layers, FFF generally has some restrictions on the slope of the overhang, and cannot produce unsupported stalactites.
Myriad materials are available, such as Acrylonitrile Butadiene Styrene (ABS), Polylactic acid (PLA), Polycarbonate (PC), Polyamide (PA), Polystyrene (PS), lignin, rubber, among many others, with different trade-offs between strength and temperature properties. In addition, even the color of a given thermoplastic material may affect the strength of the printed object. Recently a German company demonstrated for the first time the technical possibility of processing granular PEEK into filament form and 3D printing parts from the filament material using FFF technology.
During FFF, the hot molten polymer is exposed to air. Operating the FFF process within an inert gas atmosphere such as nitrogen or argon can significantly increase the layer adhesion and leads to improved mechanical properties of the 3D printed objects. An inert gas is routinely used to prevent oxidation during selective laser sintering.
Physics of the process

During extrusion the thermoplastic filament is introduced by mechanical pressure from rollers, into the liquefier (or hotend), where it melts and is then extruded. Flow geometry of the extruder, heating method and the melt flow behavior of a non-Newtonian fluid are of main consideration in the part. The rollers are the only drive mechanism in the material delivery system, therefore filament is under tensile stress upstream to the roller and under compression at the downstream side acting as a plunger. Therefore, compressive stress is the driving force behind the extrusion process.
The force required to extrude the melt must be sufficient to overcome the pressure drop across the system, which strictly depends on the viscous properties of the melted material and the flow geometry of the liquefier and nozzle. The melted material is subjected to shear deformation during the flow. Shear thinning behavior is observed in most of the materials used in this type of 3-D printing. This is modeled using power law for generalized Newtonian fluids.
The temperature is regulated by heat input from electrical coil heaters. The system continuously adjusts the power supplied to the coils according to the temperature difference between the desired value and the value detected by the thermocouple, forming a negative feedback loop. This is similar to ambient heating of a room.
Applications
Commercial applications
FFF and the other technologies of additive manufacturing by material extrusion (EAM) techniques are commonly used for prototyping and rapid manufacturing. Rapid prototyping facilitates iterative testing, and for very short runs, rapid manufacturing can be a relatively inexpensive alternative. EAM is also used in prototyping scaffolds for medical tissue engineering applications. Moreover, EAM with multi extrusion have become very popular to fabricate biomimetic composites. FFF is also applied in manufacturing within other sectors, including aerospace, automotive, construction, electronics, energy, pharmaceuticals, sports, textiles, and toys.
Free applications



There are multiple projects in the open-sourced community aimed at processing post-consumer plastic waste into filament. These involve machines used to shred and extrude the plastic material into filament such as recyclebots.
Several projects and companies are making efforts to develop affordable 3D printers for home desktop use. Much of this work has been driven by and targeted at DIY/enthusiast/early adopter communities, with additional ties to the academic and hacker communities.
RepRap is one of the longest running projects in the desktop category. The RepRap project aims to produce a free and open source hardware (FOSH) 3D printer, whose full specifications are released under the GNU General Public License, and which is capable of replicating itself by printing many of its own (plastic) parts to create more machines. RepRaps have already been shown to be able to print circuit boards and metal parts. Fab@Home is the other opensource hardware project for DIY 3D printers.
Because of the FOSH aims of RepRap, many related projects have used their design for inspiration, creating an ecosystem of related or derivative 3D printers, most of which are also open source designs. The availability of these open source designs means that variants of 3D printers are easy to invent. The quality and complexity of printer designs, however, as well as the quality of kit or finished products, varies greatly from project to project. This rapid development of open source 3D printers is gaining interest in many spheres as it enables hyper-customization and the use of public domain designs to fabricate open source appropriate technology. This technology can also assist initiatives in sustainable development since technologies are easily and economically made from resources available to local communities.
Development
Customer-driven product customization and demand for cost and time savings have increased interest in agility of manufacturing process. This has led to improvements in rapid prototyping technologies. The development of extruders is going rapidly because of the open source 3-D printer movement caused by products like RepRap. E3D and BondTech are the most known extruder manufacturers currently on the market. Consistent improvements are seen in the form of increased heating temperature of liquefiers, better control and precision of prints, and improved support for a wide variety of materials. Besides the improved hardware, the ability to calibrate the extruder according to the hardware setup has come a long way.
Cost of 3D printer
The cost of 3D printers has decreased dramatically since about 2010, with machines that used to cost US$20,000 now costing less than US$1,000. For instance, as of 2017, several companies and individuals are selling parts to build various RepRap designs, with prices starting at about £99 / US$100.
The open source Fab@Home project has developed printers for general use with anything that can be extruded through a nozzle, from chocolate to silicone sealant and chemical reactants. Printers following the project's designs have been available from suppliers in kits or in pre-assembled form since 2012 at prices in the US$2,000 range.
The LulzBot 3D printers manufactured by Aleph Objects are another example of an open-source application of fused deposition modeling technology. The flagship model in the LulzBot line, the TAZ printer takes inspiration for its design from the RepRap Mendel90 and Prusa i3 models. The LulzBot 3D printer is currently the only printer on the market to have received the "Respects Your Freedom" certification from the Free Software Foundation.
As of September 2018 RepRap style printers are readily available in kit form through online retailers. These kits come complete with all parts needed to make a functioning printer, often including electronic files for test printing as well as a small quantity of PLA filament.
Filaments used for printing with FDM printers are also substantially more cost-effective than their SLA resin counterparts. If we use 3DBenchy as a benchmark for comparing both technologies, it would cost roughly $0.20 to print such a model with an FDM machine, whereas the same object would cost almost $1.00 if created with resin.
Materials
Plastic is the most common material for 3d printing via FFF and other EAM variants. Various polymers may be used, including acrylonitrile butadiene styrene (ABS), polycarbonate (PC), polylactic acid (PLA), high-density polyethylene (HDPE), PC/ABS, polyethylene terephthalate (PETG), polyphenylsulfone (PPSU) and high impact polystyrene (HIPS). In general, the polymer is in the form of a filament fabricated from virgin resins. Additionally, fluoropolymers such as PTFE tubing are used in the process due to the material's ability to withstand high temperatures. This ability is especially useful in transferring filaments.
The many different variants of EAM, i.e. of material Extrusion based Additive Manufacturing allow dealing with many additional material types, summarised in the table below. Several material classes can be extruded and 3d printed:
- Thermoplastic polymers, it is the most typical application of FDM;
- Composite materials with polymeric matrix and short or long hard fibers;
- Ceramic slurries and clays, often used in combination with the robocasting technique;
- Green mixtures of ceramic or metal powders and polymeric binders, used in EAM of metals and ceramics;
- Food pastes;
- Biological pastes, used in bioprinting.
Material class | examples | Post-processing requirements | Typical applications |
---|---|---|---|
Thermoplastic polymers | PLA, PETG, ABS, ASA, HDPE, PPSF, PC, Ultem 9085, PEEK, recycled plastics | support removal | General purpose. These materials have varying physical properties, such as heat resistance, UV resistance, storage requirements, ease of printing, cost, and chemical tolerance. They are available in a variety of formulations to fine tune them to the specific applications (such as ESD material blends, or the addition of flame retardants). |
Polymer matrix composites | GFRP, CFRP | support removal, curing | Structural applications |
Ceramic slurries and clays | Alumina, Zirconia, Kaolin | support removal, furnace drying and sintering | Insulation, consumers objects, dental applications |
Green ceramic/binder mixture | Zirconia, Calcium phosphate | support removal, debinding, sintering | structural ceramics, piezoelectric components |
Green metal/binder mixture | Stainless steel, Titanium, Inconel | support removal, debinding, sintering | Tooling, fixtures, mechanical parts |
Green metal/ceramic/binder mixture | Stainless steel, Iron, tricalciumphosphate, yttria-stabilized zirconia | support removal, debinding, sintering | Mechanical parts, implants |
Food pastes | chocolate, sugar | support removal | |
Biological materials | bioink | bioprinted organs and scaffolds | |
Conductive polymer composites | Composites with Carbon Black, Graphene, Carbon Nano tubes or Copper Nanoparticles | Annealing for lower conductivity | Sensors |
polymer derived ceramics (PDCs) | poly lactic acid (PLA), polycarbonate (PC), nylon alloys, polypropylene (PP), polyethylene terephthalate glycol (PETG), polyethylene terephthalate (PET), and co-polyesters; and flexible materials including: flexible PLA, thermoplastic elastomer and thermoplastic polyurethane filaments | To make SiOC(N) first the printed polymer is dipped in PDC, absorbed then sintered | heat exchangers, heat sinks, scaffoldings for bone tissue growth, chemical/ gas filters and custom scientific hardware |
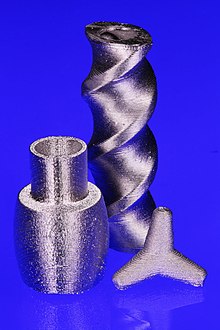

Print head kinematics
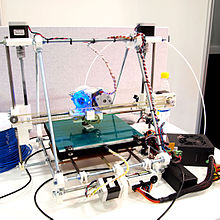
The majority of fused filament printers follow the same basic design. A flat bed is used as the starting point for the print workpiece. A gantry above this carries the moving print head. The gantry design is optimized for movement mostly in the horizontal X & Y directions, with a slow climb in the Z direction as the piece is printed. Stepper motors drive the movement through either leadscrews or toothed belt drives. It is common, owing to the differences in movement speed, to use toothed belts for the X, Y drives and a leadscrew for Z. Some machines also have X axis movement on the gantry, but move the bed (and print job) for Y. As, unlike laser cutters, head movement speeds are low, stepper motors are universally used and there is no need to use servomotors instead.
Many printers, originally those influenced by the RepRap project, make extensive use of 3D printed components in their own construction. These are typically printed connector blocks with a variety of angled holes, joined by cheap steel threaded rod. This makes a construction that is cheap and easy to assemble, easily allows non-perpendicular framing joints, but does require access to a 3D printer. The notion of 'bootstrapping' 3D printers like this has been something of a dogmatic theme within the RepRap designs. The lack of stiffness in the rod also requires either triangulation, or gives the risk of a gantry structure that flexes and vibrates in service, reducing print quality.
Many machines, especially commercial machines such as the Bambu X1, Ultimaker S Series and Creality K2, now use box-like semi-enclosed frames of either laser-cut plywood, plastic, pressed steel sheet and more recently aluminum extrusions. These are cheap, rigid and can also be used as the basis for an enclosed print volume, allowing temperature control within it to control warping of the print job.
A handful of machines use polar coordinates instead, usually machines optimized to print objects with circular symmetry. These have a radial gantry movement and a rotating bed. Although there are some potential mechanical advantages to this design for printing hollow cylinders, their different geometry and the resulting non-mainstream approach to print planning still keeps them from being popular as yet. Although it is an easy task for a robot's motion planning to convert from Cartesian to polar coordinates, gaining any advantage from this design also requires the print slicing algorithms to be aware of the rotational symmetry from the outset.
Extruder mount to rest of machine
The ways extruders are mounted on the rest of the machine have evolved over time into informal mounting standards. Such factor standards allow new extruder designs to be tested on existing printer frames, and new printer frame designs to use existing extruders. These informal standards include:
Delta robot printers

A different approach is taken with 'Rostock' or 'Kossel' pattern printers, based on a delta robot mechanism. These have a large open print volume with a three-armed delta robot mounted at the top. This design of robot is noted for its low inertia and ability for fast movement over a large volume. Stability and freedom from vibration when moving a heavy print head on the end of spindly arms is a technical challenge though. This design has mostly been favored as a means of gaining a large print volume without a large and heavy gantry.
As the print head moves the distance of its filament from storage coil to head also changes, the tension created on the filament is another technical challenge to overcome to avoid affecting the print quality.
See also
- 3D pen
- 3D printing
- Ball bearing
- Extruder (3D printing)
- Fab lab
- Fab@Home
- G-code
- Hyrel 3D
- MakerBot
- Marlin (firmware)
- Methacrylate
- Plastic extrusion
- Printrbot
- Prusa i3
- Rapid prototyping
- RepRap
- Robo 3D
- Selective laser melting
- Selective laser sintering
- Sindoh
- Spindle
- Stepper motor
- Stereolithography
- Thermistor
- Thermocouple
- Ultimaker
- Von Neumann universal constructor
References
- Hamzah, Hairul Hisham; Saiful, Arifin Shafiee; Aya, Abdalla; Patel, Bhavik Anil (2018). "3D printable conductive materials for the fabrication of electrochemical sensors: A mini review". Electrochemistry Communications. 96: 27–371. doi:10.1016/j.elecom.2018.09.006. S2CID 105586826.
- ^ Jones, R.; Haufe, P.; Sells, E.; Iravani, P.; Olliver, V.; Palmer, C.; Bowyer, A. (2011). "Reprap-- the replicating rapid prototyper". Robotica. 29 (1): 177–191. doi:10.1017/S026357471000069X.
- "A Comprehensive List of All 3D Printing Technologies". MANUFACTUR3D. 2018-11-05. Retrieved 2018-11-06.
- Bin Hamzah, Hairul Hisham; Keattch, Oliver; Covill, Derek; Patel, Bhavik Anil (2018). "The effects of printing orientation on the electrochemical behaviour of 3D printed acrylonitrile butadiene styrene (ABS)/carbon black electrodes". Scientific Reports. 8 (1): 9135. Bibcode:2018NatSR...8.9135B. doi:10.1038/s41598-018-27188-5. PMC 6002470. PMID 29904165.
- "Category:Thermoplastics". RepRap Wiki. Retrieved 2 November 2014.
- "FDM(Fused Deposition Modeling)". rpworld.net. Archived from the original on August 12, 2013. Retrieved December 27, 2017.
- Chua, Chee Kai; Leong, Kah Fai; Lim, Chu Sing (2003). Rapid Prototyping: Principles and Applications. Singapore: World Scientific. p. 124. ISBN 9789812381170.
- "Patent#:US005121329". United States Patent and Trademark Office.
- Rundle, Guy (2014). A Revolution in the Making. South Melbourne, VIC: Affirm Press. ISBN 9781922213303.
- Stratasys. "Stratasys Legal Information". stratasys.com. Retrieved 2016-07-20.
- United States Patent and Trademark Office. "Trademark Status Document Retrieval (TSDR): Registration Number 4325106". uspto.gov. Retrieved 2017-08-20.
- Gibson, I; Rosen, D W; Stucker, B (2010). Additive Manufacturing Technologies: Rapid Prototyping to Direct Digital Manufacturing. Boston, MA: Springer. ISBN 9781441911193.
- Conner, Brett P.; Manogharan, Guha P.; Martof, Ashley N.; Rodomsky, Lauren M.; Rodomsky, Caitlyn M.; Jordan, Dakesha C.; Limperos, James W. (2014). "Making sense of 3-D printing: Creating a map of additive manufacturing products and services". Addit Manuf. 1–4: 64–76. doi:10.1016/j.addma.2014.08.005.
- ^ "FDM Extruders". RepRap wiki. Retrieved 24 October 2014.
- Ciprian (4 May 2020). "How To Change The 3D Printer Nozzle?". 3D Print Beginner. Retrieved 2020-05-24.
- Bose, Animesh; Schuh, Christopher A.; Tobia, Jay C.; Tuncer, Nihan; Mykulowycz, Nicholas M.; Preston, Aaron; Barbati, Alexander C.; Kernan, Brian; Gibson, Michael A. (2018-06-01). "Traditional and additive manufacturing of a new Tungsten heavy alloy alternative". International Journal of Refractory Metals and Hard Materials. 73: 22–28. doi:10.1016/j.ijrmhm.2018.01.019. ISSN 0263-4368. S2CID 139180552.
- "Universal Paste extruder – Ceramic, Food and Real Chocolate 3D Printing". Richrap.blogspot.com. 2012-04-06. Retrieved 2 November 2014.
- Wang, Jiwen; Shaw, Leon L.; Cameron, Thomas B. (2006). "Solid Freeform Fabrication of Permanent Dental Restorations via Slurry Micro-Extrusion". Journal of the American Ceramic Society. 89 (1): 346–349. doi:10.1111/j.1551-2916.2005.00672.x. ISSN 1551-2916.
- Volpato, N.; Kretschek, D.; Foggiatto, J. A.; Gomez da Silva Cruz, C. M. (2015-12-01). "Experimental analysis of an extrusion system for additive manufacturing based on polymer pellets". The International Journal of Advanced Manufacturing Technology. 81 (9): 1519–1531. doi:10.1007/s00170-015-7300-2. ISSN 1433-3015. S2CID 110866375.
- ^ Rane, Kedarnath; Di Landro, Luca; Strano, Matteo (2019-01-06). "Processability of SS316L powder - binder mixtures for vertical extrusion and deposition on table tests". Powder Technology. 345: 553–562. doi:10.1016/j.powtec.2019.01.010. hdl:11311/1074304. ISSN 0032-5910. S2CID 104453792.
- "Xomerty Design Guide: Fused Deposition Modeling" (PDF). Hubspot.net. Xometry. Retrieved December 12, 2018.
- ^ Bellini, Anna; Güçeri, Selçuk; Bertoldi, Maurizio (2014). "Liquefier Dynamics in Fused Deposition". Journal of Manufacturing Science and Engineering. 126 (2): 237. doi:10.1115/1.1688377.
- Wittbrodt, Ben; Pearce, Joshua M. (2015-10-01). "The effects of PLA color on material properties of 3-D printed components". Additive Manufacturing. 8: 110–116. doi:10.1016/j.addma.2015.09.006.
- "PEEK being 3D-printed". 3dprint.com. March 21, 2015. Retrieved March 26, 2015.
- Lederle, Felix; Meyer, Frederick; Brunotte, Gabriella-Paula; Kaldun, Christian; Hübner, Eike G. (2016-04-19). "Improved mechanical properties of 3D-printed parts by fused deposition modeling processed under the exclusion of oxygen". Progress in Additive Manufacturing. 1 (1–2): 3–7. doi:10.1007/s40964-016-0010-y.
- Jacobson, David; Rennie, Allan; Bocking, Chris (29 September 2004). Fifth National Conference on Rapid Design, Prototyping and Manufacture. John Wiley & Sons. ISBN 9781860584657 – via Google Books.
- Melchels, Ferry; Severin Wiggenhauser, Paul; Warne, David; Barry, Mark; Ong, Fook Rhu; Chong, Woon Shin; Werner Hutmacher, Dietmar; Schantz, Jan-Thorsten (2011). "CAD/CAM-assisted breast reconstruction". Biofabrication. 3 (3): 034114. Bibcode:2011BioFa...3c4114M. doi:10.1088/1758-5082/3/3/034114. PMID 21900731. S2CID 206108959.
- Islam, Muhammed Kamrul; Hazell, Paul J.; Escobedo, Juan P.; Wang, Hongxu (July 2021). "Biomimetic armour design strategies for additive manufacturing: A review". Materials & Design. 205: 109730. doi:10.1016/j.matdes.2021.109730.
- Dash, Aparna; Kabra, Shruti; Misra, Sidhant; G, Hrishikeshan (November 2022). "Comparative property analysis of fused filament fabrication PLA using fresh and recycled feedstocks". Materials Research Express. 55 (11). Bibcode:2022MRE.....9k5303D. doi:10.1088/2053-1591/ac96d4.
- Kalish, Jon (November 28, 2010). "A Space For DIY People To Do Their Business". NPR.org. Retrieved 2012-01-31.
- "Open source 3D printer copies itself". Computerworld New Zealand. 2008-04-07. Retrieved 2013-10-30.
- "First RepRapped circuit". blog.reprap.org. April 19, 2009.
- Bhanoo, Sindya N. (9 December 2013). "An Inexpensive Way to Print Out Metal Parts". The New York Times.
- Anzalone, Gerald C.; Zhang, Chenlong; Wijnen, Bas; Sanders, Paul G.; Pearce, Joshua M. (2013). "Low-Cost Open-Source 3-D Metal Printing". IEEE Access. 1: 803–810. doi:10.1109/ACCESS.2013.2293018.
- Pearce, Joshua M.; et al. (2010). "3-D Printing of Open Source Appropriate Technologies for Self-Directed Sustainable Development". Journal of Sustainable Development. 3 (4): 17–29. CiteSeerX 10.1.1.678.781. doi:10.5539/jsd.v3n4p17. S2CID 42030603.
- "3D4D Challenge". TechForTrade.org. Archived from the original on 2014-12-27.
- "Extruder Calibration Guide (with Calculator) - E Step Calibration". 3D Print Beginner. 2020-04-14. Retrieved 2020-05-24.
- Bilton, Nick (2013-02-17). "Disruptions: On the Fast Track to Routine 3-D Printing". Bits. New York Times.
- "3D printers list with prices". 3ders.org. Retrieved 2013-10-30.
- "Desktop fabricator may kick-start home revolution". New Scientist. 9 January 2007.
- Gay, Joshua (29 Apr 2013). "Aleph Objects". fsf.org. Free Software Foundation, Inc. Retrieved 2 April 2015.
- Mayer, Martin. "SLA vs FDM printers for miniatures: pros and cons of each type – 3D Solved". Retrieved 2022-06-15.
- "Which 3D printer filament emits the most nanoparticles?". alveo3D. 25 April 2023.
- Morris, Ali (26 May 2022). "Polyformer is an open-source machine that recycles plastic bottles into 3D printing filament". Dezeen. Retrieved 26 September 2022.
- Ning, Fuda; Cong, Weilong; Qiu, Jingjing; Wei, Junhua; Wang, Shiren (2015-10-01). "Additive manufacturing of carbon fiber reinforced thermoplastic composites using fused deposition modeling". Composites Part B: Engineering. 80: 369–378. doi:10.1016/j.compositesb.2015.06.013. ISSN 1359-8368.
- Cesarano, Joseph (1998). "A Review of Robocasting Technology". MRS Online Proceedings Library Archive. 542. doi:10.1557/PROC-542-133. ISSN 1946-4274.
- Grida, Imen; Evans, Julian R. G. (2003-04-01). "Extrusion freeforming of ceramics through fine nozzles". Journal of the European Ceramic Society. 23 (5): 629–635. doi:10.1016/S0955-2219(02)00163-2. ISSN 0955-2219.
- S.B. Hein, L. Reineke, V. Reinkemeyer: Fused Filament Fabrication of Biodegradable Materials for Implants, Proceeding of Euro PM 2019 Congress & Exhibition, Maastricht 13.-16. October 2019,, European Powder Metallurgy Association EPMA, Shrewsbury, 2019, ISBN 978-1-899072-51-4.
- Sun, Jie; Zhou, Weibiao; Huang, Dejian; Fuh, Jerry Y. H.; Hong, Geok Soon (2015-08-01). "An Overview of 3D Printing Technologies for Food Fabrication". Food and Bioprocess Technology. 8 (8): 1605–1615. doi:10.1007/s11947-015-1528-6. ISSN 1935-5149. S2CID 20446103.
- Liu, Wanjun; Zhang, Yu Shrike; Heinrich, Marcel A.; Ferrari, Fabio De; Jang, Hae Lin; Bakht, Syeda Mahwish; Alvarez, Mario Moisés; Yang, Jingzhou; Li, Yi-Chen (2017). "Rapid Continuous Multimaterial Extrusion Bioprinting". Advanced Materials. 29 (3): 1604630. Bibcode:2017AdM....2904630L. doi:10.1002/adma.201604630. ISSN 1521-4095. PMC 5235978. PMID 27859710.
- Schouten, Martijn; Wolterink, Gerjan; Dijkshoorn, Alexander; Kosmas, Dimitrios; Stramigioli, Stefano; Krijnen, Gijs (2020). "A Review of Extrusion-Based 3D Printing for the Fabrication of Electro-and Biomechanical Sensors". IEEE Sensors Journal. 21 (11): 12900–12912. doi:10.1109/JSEN.2020.3042436. ISSN 1530-437X. S2CID 229660718.
- Kulkarni, Apoorv; Sorarù, Gian Domenico; Pearce, Joshua M. (2020-03-01). "Polymer-derived SiOC replica of material extrusion-based 3-D printed plastics". Additive Manufacturing. 32: 100988. arXiv:1909.02442. doi:10.1016/j.addma.2019.100988. ISSN 2214-8604. S2CID 202537657.
- "Rostock". RepRap.
- "Kossel". RepRap.
Further reading
- "Results of Make Magazine's 2015 3D Printer Shootout". Retrieved 1 June 2015.
- "Evaluation Protocol for Make Magazine's 2015 3D Printer Shootout". makezine.com. 2014-11-07. Retrieved 1 June 2015.
- Stephens, Brent; Azimia, Parham; El Orcha, Zeineb; Ramos, Tiffanie (November 2013). "Ultrafine Particle Emissions from Desktop 3D Printers". Atmospheric Environment. 79: 334–339. Bibcode:2013AtmEn..79..334S. doi:10.1016/j.atmosenv.2013.06.050.
- "How Fused Deposition Modeling Works". THRE3D.com. Archived from the original on February 21, 2014. Retrieved 7 February 2014.
- "3D Printing process and How FDM technology works". homeshop3dprinting.com (Video). Retrieved 4 June 2014.
- "RepRap project's complete list of G-code used by 3D printer's firmware". RepRap.org. Retrieved 26 August 2015.
- "FDM Technology Made Simple". chizel.io. 2018-07-16. Archived from the original on 2019-07-10. Retrieved 10 July 2019.
3D printing technologies | |
---|---|
Resin photopolymerization | |
Material extrusion | |
Powder bed binding/fusion | |
Sheet lamination | |
Directed energy deposition | |
Building printing | |
Related topics |