
The carbonate–silicate geochemical cycle, also known as the inorganic carbon cycle, describes the long-term transformation of silicate rocks to carbonate rocks by weathering and sedimentation, and the transformation of carbonate rocks back into silicate rocks by metamorphism and volcanism. Carbon dioxide is removed from the atmosphere during burial of weathered minerals and returned to the atmosphere through volcanism. On million-year time scales, the carbonate-silicate cycle is a key factor in controlling Earth's climate because it regulates carbon dioxide levels and therefore global temperature.
The rate of weathering is sensitive to factors that change how much land is exposed. These factors include sea level, topography, lithology, and vegetation changes. Furthermore, these geomorphic and chemical changes have worked in tandem with solar forcing, whether due to orbital changes or stellar evolution, to determine the global surface temperature. Additionally, the carbonate-silicate cycle has been considered a possible solution to the faint young Sun paradox.
Overview of the cycle
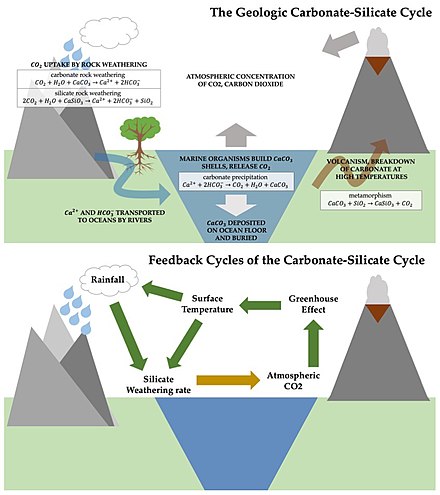
The carbonate-silicate cycle is the primary control on carbon dioxide levels over long timescales. It can be seen as a branch of the carbon cycle, which also includes the organic carbon cycle, in which biological processes convert carbon dioxide and water into organic matter and oxygen via photosynthesis.
Physical and chemical processes

Part of a series on the |
Carbon cycle |
---|
![]() |
By regions |
Carbon dioxide |
Forms of carbon
|
Metabolic pathways |
Carbon respiration |
Carbon pumps |
Carbon sequestration |
Methane |
Biogeochemical |
Other |
The inorganic cycle begins with the production of carbonic acid (H2CO3) from rainwater and gaseous carbon dioxide. Due to this process, normal rain has a pH of around 5.6. Carbonic acid is a weak acid, but over long timescales, it can dissolve silicate rocks (as well as carbonate rocks). Most of the Earth's crust (and mantle) is composed of silicates. These substances break down into dissolved ions as a result. For example, calcium silicate (CaSiO3), or wollastonite, reacts with carbon dioxide and water to yield a calcium ion, Ca, a bicarbonate ion, HCO3, and dissolved silica. This reaction structure is representative of general silicate weathering of calcium silicate minerals. The chemical pathway is as follows:
River runoff carries these products to the ocean, where marine calcifying organisms use Ca and HCO3 to build their shells and skeletons, a process called carbonate precipitation:
Two molecules of CO2 are required for silicate rock weathering; marine calcification releases one molecule back to the atmosphere. The calcium carbonate (CaCO3) contained in shells and skeletons sinks after the marine organism dies and is deposited on the ocean floor.
The final stage of the process involves the movement of the seafloor. At subduction zones, the carbonate sediments are buried and forced back into the mantle. Some carbonate may be carried deep into the mantle where high pressure and temperature conditions allow it to combine metamorphically with SiO2 to form CaSiO3 and CO2, which is released from the interior into the atmosphere via volcanism, thermal vents in the ocean, or soda springs, which are natural springs that contain carbon dioxide gas or soda water:
This final step returns the second CO2 molecule to the atmosphere and closes the inorganic carbon budget. 99.6% of all carbon on Earth (equating to roughly 10 billion tons of carbon) is sequestered in the longterm rock reservoir. And essentially all carbon has spent time in the form of carbonate. By contrast, only 0.002% of carbon exists in the biosphere.
Feedbacks
Changes to the surface of the planet, such as an absence of volcanoes or higher sea levels, which would reduce the amount of land surface exposed to weathering can change the rates at which different processes in this cycle take place. Over tens to hundreds of millions of years, carbon dioxide levels in the atmosphere may vary due to natural perturbations in the cycle but even more generally, it serves as a critical negative feedback loop between carbon dioxide levels and climate changes. For example, if CO2 builds up in the atmosphere, the greenhouse effect will serve to increase the surface temperature, which will in turn increase the rate of rainfall and silicate weathering, which will remove carbon from the atmosphere. In this way, over long timescales, the carbonate-silicate cycle has a stabilizing effect on the Earth's climate, which is why it has been called the Earth's thermostat.
Changes through Earth history
Part of a series on |
Biogeochemical cycles |
---|
![]() |
Water cycle |
Carbon cycle |
Nutrient cycle |
Rock cycle |
Marine cycle |
Methane cycle |
Other cycles |
Related topics |
Research groups |
Aspects of the carbonate-silicate cycle have changed through Earth history as a result of biological evolution and tectonic changes. Generally, the formation of carbonates has outpaced that of silicates, effectively removing carbon dioxide from the atmosphere. The advent of carbonate biomineralization near the Precambrian-Cambrian boundary would have allowed more efficient removal of weathering products from the ocean. Biological processes in soils can significantly increase weathering rates. Plants produce organic acids that increase weathering. These acids are secreted by root and mycorrhizal fungi, as well as microbial plant decay. Root respiration and oxidation of organic soil matter also produce carbon dioxide, which is converted to carbonic acid, which increases weathering.
Tectonics can induce changes in the carbonate-silicate cycle. For example, the uplift of major mountain ranges, such as Himalayas and the Andes, is thought to have initiated the Late Cenozoic Ice Age due to increased rates of silicate weathering and draw down of carbon dioxide. Seafloor weather is linked both to solar luminosity and carbon dioxide concentration. However, it presented a challenge to modelers who have tried to relate the rate of outgassing and subduction to the related rates of seafloor change. Proper, uncomplicated proxy data is difficult to attain for such questions. For example, sediment cores, from which scientists can deduce past sea levels, are not ideal because sea levels change as a result of more than just seafloor adjustment. Recent modeling studies have investigated the role of seafloor weathering on the early evolution of life, showing that relatively fast seafloor creation rates worked to draw down carbon dioxide levels to a moderate extent.
Observations of so-called deep time indicate that Earth has a relatively insensitive rock weathering feedback, allowing for large temperature swings. With about twice as much carbon dioxide in the atmosphere, paleoclimate records show that global temperatures reached up to 5 to 6 °C higher than current temperatures. However, other factors such as changes in orbital/solar forcing contribute to global temperature change in the paleo-record.
Human emissions of CO2 have been steadily increasing, and the consequent concentration of CO2 in the Earth system has reached unprecedented levels in a very short amount of time. Excess carbon in the atmosphere that is dissolved in seawater can alter the rates of carbonate-silicate cycle. Dissolved CO2 may react with water to form bicarbonate ions, HCO3, and hydrogen ions, H. These hydrogen ions quickly react with carbonate, CO3 to produce more bicarbonate ions and reduce the available carbonate ions, which presents an obstacle to the carbon carbonate precipitation process. Put differently, 30% of excess carbon emitted into the atmosphere is absorbed by the oceans. Higher concentrations of carbon dioxide in the oceans work to push the carbonate precipitation process in the opposite direction (to the left), producing less CaCO3. This process, which harms shell-building organisms, is called ocean acidification.
The cycle on other planets
One should not assume that a carbonate-silicate cycle would appear on all terrestrial planets. To begin, the carbonate-silicate cycle requires the presence of a water cycle. It therefore breaks down at the inner edge of the Solar System's habitable zone. Even if a planet starts out with liquid water on the surface, if it becomes too warm, it will undergo a runaway greenhouse, losing surface water. Without the requisite rainwater, no weathering will occur to produce carbonic acid from gaseous CO2. Furthermore, at the outer edge, CO2 may condense, consequently reducing the greenhouse effect and reducing the surface temperature. As a result, the atmosphere would collapse into polar caps.
Mars is such a planet. Located at the edge of the solar system's habitable zone, its surface is too cold for liquid water to form without a greenhouse effect. With its thin atmosphere, Mars' mean surface temperature is 210 K (−63 °C). In attempting to explain topographical features resembling fluvial channels, despite seemingly insufficient incoming solar radiation, some have suggested that a cycle similar to Earth's carbonate-silicate cycle could have existed – similar to a retreat from Snowball Earth periods. It has been shown using modeling studies that gaseous CO2 and H2O acting as greenhouse gases could not have kept Mars warm during its early history when the Sun was fainter because CO2 would condense out into clouds. Even though CO2 clouds do not reflect in the same way that water clouds do on Earth, it could not have had much of a carbonate-silicate cycle in the past.
By contrast, Venus is located at the inner edge of the habitable zone and has a mean surface temperature of 737 K (464 °C). After losing its water by photodissociation and hydrogen escape, Venus stopped removing carbon dioxide from its atmosphere, and began instead to build it up, and experience a runaway greenhouse effect.
On tidally locked exoplanets, the location of the substellar point will dictate the release of carbon dioxide from the lithosphere.
See also
- Carbon cycle
- Reverse weathering
- Daisyworld
- Gaia hypothesis
- Runaway greenhouse effect
- Major ice ages
- Snowball earth
- Lake Nyos
- Ocean acidification
- Faint young Sun paradox
References
- Urey, H. C. (1952). The planets: their origin and development. Mrs. Hepsa Ely Silliman Memorial Lectures.
- ^ Berner, Robert; Lasaga, Antonio; Garrels, Robert (1983). "The carbonate-silicate geochemical cycle and its effect on atmospheric carbon dioxide over the past 100 million years". American Journal of Science. 283 (7): 641–683. Bibcode:1983AmJS..283..641B. doi:10.2475/ajs.283.7.641.
- ^ Walker, James C. G.; Hays, P. B.; Kasting, J. F. (1981). "A negative feedback mechanism for the long-term stabilization of Earth's surface temperature". Journal of Geophysical Research: Oceans. 86 (C10): 9776–9782. Bibcode:1981JGR....86.9776W. doi:10.1029/JC086iC10p09776. ISSN 2156-2202.
- Walker, James C. G. (1993). "Biogeochemical Cycles of Carbon on a Hierarchy of Timescales". Biogeochemistry of Global Change: Radiatively Active Trace Gases Selected Papers from the Tenth International Symposium on Environmental Biogeochemistry. Boston, MA: Springer. pp. 3–28. doi:10.1007/978-1-4615-2812-8_1. ISBN 978-1-4613-6215-9.
- ^ Sullivan, Woodruff T.; Baross, John A. (2007). "Planetary Atmospheres and Life". Planets and Life. Cambridge, UK: Cambridge University Press. pp. 91–116. ISBN 978-0-521-53102-3.
- ^ Bonan, Gordon (2013). Ecological Climatology: Concepts and Applications (2nd ed.). New York: Cambridge University Press. pp. 105–128. ISBN 978-0-521-69319-6.
- Environmental Protection Agency (9 February 2016). "What is Acid Rain?".
- ^ "Geology and Climate: ACS Climate Science Toolkit". American Chemical Society.
- ^ Catling, David C.; Kasting, James F. (2017). Atmospheric Evolution on Inhabited and Lifeless Worlds. Cambridge, UK: Cambridge University Press. pp. 299–326. ISBN 978-0-521-84412-3.
- Berner, Robert A. (April 1, 1991). "A model for atmospheric CO2 over Phanerozoic time". American Journal of Science. 291 (4): 339–376. Bibcode:1991AmJS..291..339B. doi:10.2475/ajs.291.4.339.
- Berner, Robert A. (January 29, 1998). "The carbon cycle and carbon dioxide over Phanerozoic time: the role of land plants". Philosophical Transactions of the Royal Society of London. Series B: Biological Sciences. 353 (1365): 75–82. doi:10.1098/rstb.1998.0192. PMC 1692179.
- Berner, Robert A.; Beerling, David J.; Dudley, Robert; Robinson, Jennifer M.; Wildman, Jr., Richard A. (2003). "Phanerozoic Atmospheric Oxygen". Annual Review of Earth and Planetary Sciences. 31 (31): 105–134. Bibcode:2003AREPS..31..105B. doi:10.1146/annurev.earth.31.100901.141329.
- DiVenere, Vic. "The Carbon Cycle and Earth's Climate". Columbia University.
- Penman, Donald E.; Caves Rugenstein, Jeremy K.; Ibarra, Daniel E.; Winnick, Matthew J. (October 2020). "Silicate weathering as a feedback and forcing in Earth's climate and carbon cycle". Earth-Science Reviews. 209: 103298. doi:10.1016/j.earscirev.2020.103298. Retrieved 30 October 2024 – via Elsevier Science Direct.
- Ridgewell, A; Zeebe, R (2005). "The role of the global carbonate cycle in the regulation and evolution of the Earth system". Earth and Planetary Science Letters. 234 (3–4): 299–315. doi:10.1016/j.epsl.2005.03.006. ISSN 0012-821X.
- Taylor, Lyla L.; Banwart, Steve A.; Valdes, Paul J.; Leake, Jonathan R.; Beerling, David J. (2012). "Evaluating the effects of terrestrial ecosystems, climate and carbon dioxide on weathering over geological time: a global-scale process-based approach". Philosophical Transactions of the Royal Society B: Biological Sciences. 367 (1588): 565–582. doi:10.1098/rstb.2011.0251. ISSN 0962-8436. PMC 3248708. PMID 22232768.
- Berner, Robert A. (1992). "Weathering, plants, and the long-term carbon cycle". Geochimica et Cosmochimica Acta. 56 (8): 3225–3231. Bibcode:1992GeCoA..56.3225B. doi:10.1016/0016-7037(92)90300-8. ISSN 0016-7037.
- Raymo, Maureen E.; Ruddiman, William F.; Froelich, Philip N. (1988). "Influence of late Cenozoic mountain building on ocean geochemical cycles". Geology. 16 (7): 649. Bibcode:1988Geo....16..649R. doi:10.1130/0091-7613(1988)016<0649:iolcmb>2.3.co;2. ISSN 0091-7613.
- Brady, Patrick; Gíslason, Sigurdur R. (March 1997). "Seafloor weathering controls on atmospheric CO2 and global climate Author links open overlay panel". Geochimica et Cosmochimica Acta. 61 (5): 965–973. doi:10.1016/S0016-7037(96)00385-7.
- Berner, Robert A.; Lasaga, Antonio C. (March 1989). "Modeling the Geochemical Carbon Cycle". Scientific American. 260 (3): 74–81. Bibcode:1989SciAm.260c..74B. doi:10.1038/scientificamerican0389-74.
- Krissansen-Totton, Joshua; Arney, Giada N.; Catling, David C. (April 17, 2018). "Constraining the climate and ocean pH of the early Earth with a geological carbon cycle model". PNAS. 115 (16): 4105–4110. arXiv:1804.00763. Bibcode:2018PNAS..115.4105K. doi:10.1073/pnas.1721296115. PMC 5910859. PMID 29610313.
- Krissansen-Totton, Joshua; Catling, David C. (22 May 2017). "Constraining climate sensitivity and continental versus seafloor weathering using an inverse geological carbon cycle model". Nature Communications. 8: 15423. Bibcode:2017NatCo...815423K. doi:10.1038/ncomms15423. PMC 5458154. PMID 28530231.
- Core Writing Team; R.K. Pachauri; L.A. Meyer, eds. (2014). IPCC, 2014: Climate Change 2014: Synthesis Report. Contribution of Working Groups I, II and III to the Fifth Assessment Report of the Intergovernmental Panel on Climate Change. Geneva, Switzerland: IPCC.
- "Ocean Acidification". Ocean Carbon and Biogeochemistry. Woods Hole Oceanographic Institute (WHOI).
- "Carbon Cycle". Earth Observatory. NASA. 2011-06-16.
- Batalha, Natasha E.; Kopparapu, Ravi Kumar; Haqq-Misra, Jacob; Kasting, James F. (2016). "Climate cycling on early Mars caused by the carbonate-silicate cycle". Earth and Planetary Science Letters. 455: 7–13. arXiv:1609.00602. Bibcode:2016E&PSL.455....7B. doi:10.1016/j.epsl.2016.08.044. S2CID 119257332.
- Kasting, J. F. (1991). "CO2 condensation and the climate of early Mars". Icarus. 94 (1): 1–13. Bibcode:1991Icar...94....1K. doi:10.1016/0019-1035(91)90137-I. PMID 11538088.
- Forget, François; Pierrehumbert, Raymond T. (1997). "Warming Early Mars with Carbon Dioxide Clouds that Scatter Infrared Radiation". Science. 278 (5341): 1273–1276. Bibcode:1997Sci...278.1273F. doi:10.1126/science.278.5341.1273. PMID 9360920.
- Edson, Adam R.; Kasting, James F.; Pollard, David; Lee, Sukyoung; Bannon, Peter R. (2012). "The Carbonate-Silicate Cycle and CO2/Climate Feedbacks on Tidally Locked Terrestrial Planets". Astrobiology. 12 (6): 562–571. Bibcode:2012AsBio..12..562E. doi:10.1089/ast.2011.0762. ISSN 1531-1074. PMID 22775488.
External links
- Understanding the long-term carbon-cycle: Weathering of rocks – a vitally important carbon-sink by John Mason, Skeptical Science
Biogeochemical cycles | |
---|---|
Cycles | |
Research groups | |
Related topics | |