Targeted covalent inhibitors (TCIs) or Targeted covalent drugs are rationally designed inhibitors that bind and then bond to their target proteins. These inhibitors possess a bond-forming functional group of low chemical reactivity that, following binding to the target protein, is positioned to react rapidly with a proximate nucleophilic residue at the target site to form a bond.
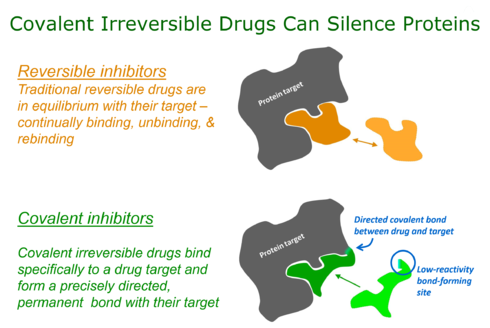
Historical impact of covalent drugs
Over the last 100 years covalent drugs have made a major impact on human health and have been highly successful drugs for the pharmaceutical industry. These inhibitors react with their target proteins to form a covalent complex in which the protein has lost its function. The majority of these successful drugs, which include penicillin, omeprazole, clopidogrel, and aspirin were discovered through serendipity in phenotypic screens.

However, key changes in screening approaches, along with safety concerns, have made pharma reluctant to pursue covalent inhibitors in a systematic way (Liebler & Guengerich, 2005). Recently, there has been considerable attention to using rational drug design to create highly selective covalent inhibitors called targeted covalent inhibitors. The first published example of a targeted covalent drug was for the EGFR kinase. But this has now broadened to other kinases and other protein families. Aside from small molecules, covalent probes are also being derived from peptides or proteins. By incorporation of a reactive group into a binding peptide or protein via posttranslational chemical modification or as an unnatural amino acid, a target protein can be conjugated specifically via proximity-induced reaction.
Advantages of covalent drugs
Potency
Covalent bonding can lead to potencies and ligand efficiencies that are either exceptionally high or, for irreversible covalent interactions, even essentially infinite. Covalent bonding thus allows high potency to be routinely achieved in compounds of low molecular mass, along with all the beneficial pharmaceutical properties that are associated with small size.
Selectivity
Covalent inhibitors can be designed to target a nucleophile that is unique or rare across a protein family. Thereby ensuring that covalent bond formation cannot occur with most other family members. This approach can lead to high selectivity against closely related proteins because although the inhibitor might bind transiently to the active sites of such proteins, it will not covalently label them if they lack the targeted nucleophilic residue in the appropriate position.
Pharmacodynamics
The restoration of pharmacological activity after covalent irreversible inhibition requires re-synthesis of the protein target. This has important and potentially advantageous consequences for drug pharmacodynamics in which the level and frequency of dosing relates to the extent and duration of the resulting pharmacological effect.
Built-in-biomarker
Covalent inhibitors can be used to assess target engagement which can sometimes be used pre-clinically and clinically to assess the relationship between dose of drug and efficacy or toxicity. This approach was used for covalent Btk inhibitors pre-clinically and clinically to understand the relationship between dose administered and efficacy in animal models of arthritis and target occupancy in a clinical study of healthy volunteers.
Design of covalent drugs
The design of covalent drugs requires careful optimization of both the non-covalent binding affinity (which is reflected in Ki) and the reactivity of the electrophilic warhead (which is reflected in k2).

The initial design of TCIs involves three key steps. First, bioinformatics analysis is used to identify a nucleophilic amino acid (for example, cysteine) that is either inside or near to a functionally relevant binding site on a drug target, but is rare in that protein family. Next, a reversible inhibitor is identified for which the binding mode is known. Finally, structure-based computational methods are used to guide the design of modified ligands that have electrophilic functionality, and are positioned to react specifically with the nucleophilic amino acid in the target protein.

Targeted covalent photoisomerizable ligands (photoswitches) have been developed to remotely and reversibly control the activity of receptor proteins with light. They have been used as molecular prostheses to restore visual input in the retina and auditory input in the cochlea via glutamate receptors. Ligand conjugation is targeted to specific lysine residues via an affinity labeling mechanism.
Toxicity risks associated with covalent modification of proteins
There has been a reluctance for modern drug discovery programs to consider covalent inhibitors due to toxicity concerns. An important contributor has been the drug toxicities of several high-profile drugs believed to be caused by metabolic activation of reversible drugs. For example, high dose acetaminophen can lead to the formation of the reactive metabolite N-acetyl-p-benzoquinone imine. Also, covalent inhibitors such as beta lactam antibiotics which contain weak electrophiles can lead to idiosyncratic toxicities (IDT) in some patients. It has been noted that many approved covalent inhibitors have been used safely for decades with no observed idiosyncratic toxicity. Also, that IDTs are not limited to proteins with a covalent mechanism of action. A recent analysis has noted that the risk of idiosyncratic toxicities may be mitigated through lower doses of administered drug. Doses of less than 10 mg per day rarely lead to IDT irrespective of the drug mechanism.
TCIs in clinical development
Despite the apparent lack of attention towards covalent inhibitor drug discovery by most pharmaceutical companies, there are several examples of covalent drugs that have been approved or are progressing to late-stage clinical development.
KRAS and lung, colorectal cancer
AMG 510 by Amgen is a KRAS p.G12C covalent inhibitor that has recently finished Phase I clinical trial. The drug elicited partial responses in half of evaluable patients with KRAS G12C-mutant non–small cell lung cancer, and led to stable disease in most evaluable patients with colorectal (or appendix) cancer.
EGFR and lung cancer
The second generation EGFR inhibitors Afatinib and Mobocertinib have been approved for the treatment of EGFR driven lung cancer and Dacomitinib is in late stage clinical testing. The third generation EGFR inhibitors which target mutant EGFR which is specific to the tumor but are selective against wild-type EGFR that are expected to lead to a wider therapeutic index.
ErbB family and breast cancer
The pan-ErbB inhibitor Neratinib was approved in the US in 2017 and in the EU in 2018 for the extended adjuvant treatment of adult patients with early-stage HER2-overexpressed/amplified breast cancer after trastuzumab-based therapy.
Btk and leukemia
Ibrutinib, a covalent inhibitor of Bruton's tyrosine kinase, has been approved for the treatment of chronic lymphocytic leukemia, waldenstrom’s macroglobulinemia and mantle cell lymphoma.
SARS-CoV-2 protease and COVID-19
Paxlovid is a covalent inhibitor of the 3CLpro (Mpro) enzyme. It is in Phase III trials for the early treatment of SARS-CoV-2 infected patients who have not progressed to severe COVID-19 disease, and who do not immediately require hospitalisation.
References
- ^ Singh J, Petter RC, Baillie TA, Whitty A (April 2011). "The resurgence of covalent drugs". Nature Reviews. Drug Discovery. 10 (4): 307–317. doi:10.1038/nrd3410. PMID 21455239. S2CID 5819338.
- Robertson JG (April 2005). "Mechanistic basis of enzyme-targeted drugs". Biochemistry. 44 (15): 5561–5571. doi:10.1021/bi050247e. PMID 15823014.
- Potashman MH, Duggan ME (March 2009). "Covalent modifiers: an orthogonal approach to drug design". Journal of Medicinal Chemistry. 52 (5): 1231–1246. doi:10.1021/jm8008597. PMID 19203292.
- Liebler DC, Guengerich FP (May 2005). "Elucidating mechanisms of drug-induced toxicity". Nature Reviews. Drug Discovery. 4 (5): 410–420. doi:10.1038/nrd1720. PMID 15864270. S2CID 6791573.
- ^ Park BK, Boobis A, Clarke S, Goldring CE, Jones D, Kenna JG, et al. (April 2011). "Managing the challenge of chemically reactive metabolites in drug development". Nature Reviews. Drug Discovery. 10 (4): 292–306. doi:10.1038/nrd3408. PMID 21455238. S2CID 29283248.
- ^ Singh J, Petter RC, Kluge AF (August 2010). "Targeted covalent drugs of the kinase family". Current Opinion in Chemical Biology. 14 (4): 475–480. doi:10.1016/j.cbpa.2010.06.168. PMID 20609616.
- ^ Singh J, Dobrusin EM, Fry DW, Haske T, Whitty A, McNamara DJ (March 1997). "Structure-based design of a potent, selective, and irreversible inhibitor of the catalytic domain of the erbB receptor subfamily of protein tyrosine kinases". Journal of Medicinal Chemistry. 40 (7): 1130–1135. doi:10.1021/jm960380s. PMID 9089334.
- Fry DW, Bridges AJ, Denny WA, Doherty A, Greis KD, Hicks JL, et al. (September 1998). "Specific, irreversible inactivation of the epidermal growth factor receptor and erbB2, by a new class of tyrosine kinase inhibitor". Proceedings of the National Academy of Sciences of the United States of America. 95 (20): 12022–12027. Bibcode:1998PNAS...9512022F. doi:10.1073/pnas.95.20.12022. PMC 21758. PMID 9751783.
- ^ Cohen MS, Zhang C, Shokat KM, Taunton J (May 2005). "Structural bioinformatics-based design of selective, irreversible kinase inhibitors". Science. 308 (5726): 1318–1321. doi:10.1126/science1108367. PMC 3641834. PMID 15919995.
- Hagel M, Niu D, St Martin T, Sheets MP, Qiao L, Bernard H, et al. (January 2011). "Selective irreversible inhibition of a protease by targeting a noncatalytic cysteine". Nature Chemical Biology. 7 (1): 22–24. doi:10.1038/nchembio.492. PMID 21113170.
- Ostrem JM, Peters U, Sos ML, Wells JA, Shokat KM (November 2013). "K-Ras(G12C) inhibitors allosterically control GTP affinity and effector interactions". Nature. 503 (7477): 548–551. Bibcode:2013Natur.503..548O. doi:10.1038/nature12796. PMC 4274051. PMID 24256730.
- Holm L, Moody P, Howarth M (November 2009). "Electrophilic affibodies forming covalent bonds to protein targets". The Journal of Biological Chemistry. 284 (47): 32906–32913. doi:10.1074/jbc.M109.034322. PMC 2781706. PMID 19759009.
- Xiang Z, Ren H, Hu YS, Coin I, Wei J, Cang H, Wang L (September 2013). "Adding an unnatural covalent bond to proteins through proximity-enhanced bioreactivity". Nature Methods. 10 (9): 885–888. doi:10.1038/nmeth.2595. PMC 3882359. PMID 23913257.
- Smith AJ, Zhang X, Leach AG, Houk KN (January 2009). "Beyond picomolar affinities: quantitative aspects of noncovalent and covalent binding of drugs to proteins". Journal of Medicinal Chemistry. 52 (2): 225–233. doi:10.1021/jm800498e. PMC 2646787. PMID 19053779.
- Srinivasan B (March 2021). "Explicit Treatment of Non-Michaelis-Menten and Atypical Kinetics in Early Drug Discovery*". ChemMedChem. 16 (6): 899–918. doi:10.1002/cmdc.202000791. PMID 33231926. S2CID 227157473.
- Liu Q, Sabnis Y, Zhao Z, Zhang T, Buhrlage SJ, Jones LH, Gray NS (February 2013). "Developing irreversible inhibitors of the protein kinase cysteinome". Chemistry & Biology. 20 (2): 146–159. doi:10.1016/j.chembiol.2012.12.006. PMC 3583020. PMID 23438744.
- ^ Durham TB, Blanco MJ (March 2015). "Target engagement in lead generation". Bioorganic & Medicinal Chemistry Letters. 25 (5): 998–1008. doi:10.1016/j.bmcl.2014.12.076. PMID 25630223.
- Advani RH, Buggy JJ, Sharman JP, Smith SM, Boyd TE, Grant B, et al. (January 2013). "Bruton tyrosine kinase inhibitor ibrutinib (PCI-32765) has significant activity in patients with relapsed/refractory B-cell malignancies". Journal of Clinical Oncology. 31 (1): 88–94. doi:10.1200/JCO.2012.42.7906. PMC 5505166. PMID 23045577.
- Izquierdo-Serra M, Bautista-Barrufet A, Trapero A, Garrido-Charles A, Díaz-Tahoces A, Camarero N, et al. (July 2016). "Optical control of endogenous receptors and cellular excitability using targeted covalent photoswitches". Nature Communications. 7 (1): 12221. Bibcode:2016NatCo...712221I. doi:10.1038/ncomms12221. PMC 4961765. PMID 27436051.
- Garrido-Charles A, Huet A, Matera C, Thirumalai A, Hernando J, Llebaria A, et al. (June 2022). "Fast Photoswitchable Molecular Prosthetics Control Neuronal Activity in the Cochlea". Journal of the American Chemical Society. 144 (21): 9229–9239. bioRxiv 10.1101/2021.05.25.445123. doi:10.1021/jacs.1c12314. PMC 9164239. PMID 35584208.
- Bauer RA (September 2015). "Covalent inhibitors in drug discovery: from accidental discoveries to avoided liabilities and designed therapies". Drug Discovery Today. 20 (9): 1061–1073. doi:10.1016/j.drudis.2015.05.005. PMID 26002380.
- Nakayama S, Atsumi R, Takakusa H, Kobayashi Y, Kurihara A, Nagai Y, et al. (September 2009). "A zone classification system for risk assessment of idiosyncratic drug toxicity using daily dose and covalent binding". Drug Metabolism and Disposition. 37 (9): 1970–1977. doi:10.1124/dmd.109.027797. PMID 19487250. S2CID 16997956.
- Clinical trial number NCT03600883 for "A Phase 1/2, Study Evaluating the Safety, Tolerability, PK, and Efficacy of AMG 510 in Subjects With Solid Tumors With a Specific KRAS Mutation." at ClinicalTrials.gov
- Tan CS, Kumarakulasinghe NB, Huang YQ, Ang YL, Choo JR, Goh BC, Soo RA (February 2018). "Third generation EGFR TKIs: current data and future directions". Molecular Cancer. 17 (1): 29. doi:10.1186/s12943-018-0778-0. PMC 5817792. PMID 29455654.
- "Nerlynx (neratinib maleate) Tablets". U.S. Food and Drug Administration (FDA). 21 August 2017. Retrieved 14 July 2021.
- "Nerlynx EPAR". European Medicines Agency (EMA). 17 September 2018. Retrieved 14 July 2021.
External links
Media related to Targeted covalent inhibitors at Wikimedia Commons
- Covalent drugs go from fringe field to fashionable endeavor, Chemical & Engineering News, November 9, 2020