This is an old revision of this page, as edited by ST11 (talk | contribs) at 02:35, 11 July 2012 (move links to first use). The present address (URL) is a permanent link to this revision, which may differ significantly from the current revision.
Revision as of 02:35, 11 July 2012 by ST11 (talk | contribs) (move links to first use)(diff) ← Previous revision | Latest revision (diff) | Newer revision → (diff)This article is about the table used in chemistry. For other uses, see The Periodic Table.

The periodic table is a tabular display of the chemical elements, organized on the basis of their atomic numbers and chemical properties. Elements are presented in increasing atomic number. The main body of the table is a 18 × 7 grid, and elements with the same number of valence electrons are kept together in groups, such as the halogens and the noble gases. Due to this, there are gaps that form four distinct rectangular areas or blocks. The f-block is not included in the main table, but rather is usually floated below, as an inline f-block would make the table impractically wide. Using periodic trends, the periodic table can help predict the properties of various elements and the relations between properties. As a result, it provides a useful framework for analyzing chemical behavior, and is widely used in chemistry and other sciences.
Although precursors exist, the current table is generally credited to Dmitri Mendeleev, who developed it in 1869 to illustrate periodic trends in the properties of the then-known elements; the layout has been refined and extended as new elements have been discovered and new theoretical models developed to explain chemical behavior. Mendeleev's presentation also predicted some properties of then-unknown elements expected to fill gaps in his arrangement; most of these predictions were proved correct when those elements were discovered and found to have properties close to the predictions.
All elements from atomic numbers 1 (hydrogen) to 118 (ununoctium) have been synthesized. Of these, all up to and including californium exist naturally; the rest have only been artificially synthesised in laboratories, along with numerous synthetic radionuclides of naturally occurring elements. Production of elements beyond ununoctium is being pursued, with the question of how the periodic table may need to be modified to accommodate these elements being a matter of ongoing debate.
History
Main article: History of the periodic tableFirst attempts of systemization
In 1789, Antoine Lavoisier published a list of 33 chemical elements. Although Lavoisier grouped the elements into gases, metals, nonmetals, and earths, chemists spent the following century searching for a more precise classification scheme. In 1829, Johann Wolfgang Döbereiner observed that many of the elements could be grouped into triads (groups of three) based on their chemical properties. Lithium, sodium, and potassium, for example, were grouped together as soft, reactive metals. Döbereiner also observed that, when arranged by atomic weight, the second member of each triad was roughly the average of the first and the third. This became known as the Law of Triads. German chemist Leopold Gmelin worked with this system, and by 1843 he had identified ten triads, three groups of four, and one group of five. Jean-Baptiste Dumas published work in 1857 describing relationships between various groups of metals. Although various chemists were able to identify relationships between small groups of elements, they had yet to build one scheme that encompassed them all.
German chemist August Kekulé had observed in 1858 that carbon has a tendency to bond with other elements in a ratio of one to four. Methane, for example, has one carbon atom and four hydrogen atoms. This concept eventually became known as valency. In 1864, fellow German chemist Julius Lothar Meyer published a table of the 49 known elements arranged by valency. The table revealed that elements with similar properties often shared the same valency.
English chemist John Newlands produced a series of papers in 1864 and 1865 that described his own classification of the elements: He noted that when listed in order of increasing atomic weight, similar physical and chemical properties recurred at intervals of eight, which he likened to the octaves of music. This Law of Octaves, however, was ridiculed by his contemporaries, and the Chemical Society refused to publish his work. Nonetheless, Newlands was able to draft an atomic table and use it to predict the existence of missing elements, such as germanium. The Chemical Society only acknowledged the significance of his discoveries some five years after they credited Mendeleev.
Mendeleev's table

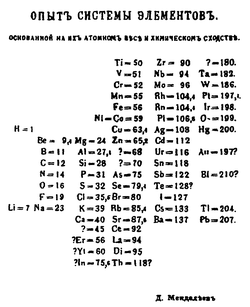
Russian chemistry professor Dmitri Ivanovich Mendeleev and German chemist Julius Lothar Meyer independently published their periodic tables in 1869 and 1870, respectively. They both constructed their tables in a similar manner: By listing the elements in a row or column in order of atomic weight and starting a new row or column when the characteristics of the elements began to repeat. The success of Mendeleev's table came from two decisions he made: The first was to leave gaps in the table when it seemed that the corresponding element had not yet been discovered. Mendeleev was not the first chemist to do so, but he was the first to be recognized as using the trends in his periodic table to predict the properties of those missing elements, such as gallium and germanium. The second decision was to occasionally ignore the order suggested by the atomic weights and switch adjacent elements, such as cobalt and nickel, to better classify them into chemical families. With the development of theories of atomic structure, it became apparent that Mendeleev had listed the elements in order of increasing atomic number.
Further development
In the years following publication of Mendeleev's periodic table, the gaps he identified were filled as chemists discovered additional naturally occurring elements. It is often stated that the last naturally occurring element to be discovered was francium (referred to by Mendeleev as eka-caesium) in 1939. However, plutonium, produced synthetically in 1940, was identified in trace quantities as a naturally occurring primordial element in 1971, and in 2011 it was found that all the elements up to californium can occur naturally in trace amounts in uranium ores.
With the development of modern quantum mechanical theories of electron configurations within atoms, it became apparent that each row (or period) in the table corresponded to the filling of a quantum shell of electrons. In Mendeleev's original table, each period was the same length. However, because larger atoms have more electron sub-shells, modern tables have progressively longer periods further down the table.
Previously, the groups were known by roman numerals. In America, the roman numerals were followed by either an "A" if the group was in the s- or p-block, or a "B" if the group was in the d-block. The roman numerals used correspond to the last digit of today's naming convention (e.g. the group 4 elements were group IVB, and the carbon group were group IVA). In Europe, the lettering was similar, except that "A" was used if the group was before group 10, and "B" was used for groups including and after group 10. In addition, groups 8, 9 and 10 used to be treated as one triple-sized group, known collectively in both notations as group VIII. In 1988, the new IUPAC naming system was put into use, and the old group names were deprecated.
The production of various transuranic elements has expanded the periodic table significantly, the first of these being neptunium, synthesized in 1939. Because many of the transuranic elements are highly unstable and decay quickly, they are challenging to detect and characterize when produced, and there have been controversies concerning the acceptance of competing discovery claims for some elements, requiring independent review to determine which party has priority, and hence naming rights. The most recently accepted and named elements are flerovium (114) and livermorium (116), both named on 31 May 2012. In 2010, a joint Russia–US collaboration at Dubna, Moscow Oblast, Russia, claimed to have synthesized six atoms of ununseptium, making it the most recently claimed discovery.
Contents of the periodic table
For a larger version, see Periodic table (large version). Periodic table Primordial From decay Synthetic Border shows natural occurrence of the element Standard atomic weight Ar, std(E)- Ca: 40.078 — Abridged value (uncertainty omitted here)
- Po: — mass number of the most stable isotope
s-block | f-block | d-block | p-block |
All versions of the periodic table only include chemical elements, not mixtures, compounds, or subatomic particles, and each isotope of a given element is represented in the same cell. In the standard periodic table, the elements are listed in order of increasing atomic number (the number of protons in the nucleus of an atom). A new row (period) is started when a new electron shell#shell has its first electron. Columns (groups) are determined by the electron configuration of the atom; elements with the same number of electrons in a particular subshell fall into the same columns (e.g. oxygen and selenium are in the same column because they both have 4 electrons in the outermost p-subshell). The periods are longer further down in the periodic table, and the groups get longer on the right (although the alkali metals, the largest group, is on the far left, and the alkaline earth metals, another large group, are next to the alkali metals). In general, elements with similar chemical properties fall into the same group in the periodic table, although in the f-block, and to some respect in the d-block, the elements in the same period tend to have similar properties, as well. Thus, it is relatively easy to predict the chemical properties of an element if one knows the properties of the elements around it.
As of 2012, the periodic table contains 118 confirmed chemical elements. Of these elements, 114 have been officially recognized and named by the International Union of Pure and Applied Chemistry (IUPAC). A total of 98 of these occur naturally, of which 84 are primordial. The other 14 elements only occur in decay chains of primordial elements. All elements from einsteinium to copernicium, as well as flerovium, and livermorium, while not occurring naturally in the universe, have been officially recognized by the IUPAC as being synthesized, while elements 113, 115, 117 and 118 have reportedly been synthesized in laboratories and are currently known only by their systematic element names, based off their atomic numbers. No element heavier than einsteinium (element 99) has ever been observed in macroscopic quantities in its pure form. No elements past 118 have been synthesized as of 2012.
In printed or other formally presented periodic tables, each element is provided a formatted cell that usually provides some of the basic properties of the element. Atomic number, element symbol, and name are almost always included, and atomic weights, densities, melting and boiling points, crystal structure as a solid, origin, abbreviated electron configuration, electronegativity, and most common valence numbers are sometimes included as well.
By definition, each chemical element has a unique atomic number representing the number of protons in its nucleus, but most elements have differing numbers of neutrons among different atoms; these are referred to as isotopes. For example, all atoms of carbon have six protons and usually have six neutrons as well, but about 1% have seven neutrons, and a very small amount have eight neutrons; so carbon has three different naturally occurring isotopes. Isotopes are never separated in the periodic table; they are always grouped together under a single element. Elements with no stable isotopes have the atomic masses of their most stable isotopes listed in parentheses.
Organization
In the modern periodic table, the elements are placed progressively in each period from left to right in the sequence of their atomic numbers, with a new row started after a noble gas. The first element in the next row is always an alkali metal with an atomic number one greater than that of the noble gas (e.g. after krypton, a noble gas with the atomic number 36, a new row is started by rubidium, an alkali metal with the atomic number 37). No gaps currently exist because all elements between hydrogen and ununoctium (element 118) have been discovered. Since the elements are sequenced by atomic number, sets of elements are sometimes specified by terms such as "through" (e.g. through iron), "beyond" (e.g. beyond uranium), or "from ... through" (e.g. from lanthanum through lutetium). The terms "light" and "heavy" are sometimes also used informally to indicate relative atomic numbers (not densities), as in "lighter than carbon" or "heavier than lead", although technically the weight or mass of atoms of an element (their atomic weights or atomic masses) do not always increase monotonically with their atomic numbers. For instance tellurium, element 52, is on average heavier than iodine, element 53.
Hydrogen and helium are often placed in different places than their electron configurations would indicate; Hydrogen is usually placed above lithium, in accordance with its electron configuration, but is sometimes placed above fluorine, or even carbon, as it also behaves similarly to them. Helium is almost always placed above neon, as they are very similar chemically.
The significance of atomic numbers to the organization of the periodic table was not appreciated until the existence and properties of protons and neutrons became understood. Mendeleev's periodic tables instead used atomic weights, information determinable to fair precision in his time, which worked well enough in most cases to give a powerfully predictive presentation far better than any other comprehensive portrayal of the chemical elements' properties then possible. Substitution of atomic numbers, once understood, gave a definitive, integer-based sequence for the elements, still used today even as new synthetic elements are being produced and studied.
Grouping methods
Groups
Main article: Group (periodic table)A group or family is a vertical column in the periodic table. Groups are considered the most important method of classifying the elements. In some groups, the elements have very similar properties and exhibit a clear trend in properties down the group. Under the international naming system, the groups are numbered numerically from 1 to 18 from the leftmost column (the alkali metals) to the rightmost column (the noble gases). The older naming systems differed slightly between Europe and America.
Some of these groups have been given trivial (unsystematic) names, such as the alkali metals, alkaline earth metals, pnictogens, chalcogens, halogens, and noble gases. However, some other groups, such as group 4, have no trivial names and are referred to simply by their group numbers, since they display fewer similarities and/or vertical trends.
Modern quantum mechanical theories of atomic structure explain group trends by proposing that elements within the same group generally have the same electron configurations in their valence shell, which is the most important factor in accounting for their similar properties.
Elements in the same group show patterns in atomic radius, ionization energy, and electronegativity. From top to bottom in a group, the atomic radii of the elements increase. Since there are more filled energy levels, valence electrons are found farther from the nucleus. From the top, each successive element has a lower ionization energy because it is easier to remove an electron since the atoms are less tightly bound. Similarly, a group has a top to bottom decrease in electronegativity due to an increasing distance between valence electrons and the nucleus.
Periods
Main article: Period (periodic table)A period or series is a horizontal row in the periodic table. Although groups are the most common way of classifying elements, there are regions where horizontal trends are more significant than vertical group trends, such as the f-block, where the lanthanides and actinides form two substantial horizontal series of elements.
Elements in the same period show trends in atomic radius, ionization energy, electron affinity, and electronegativity. Moving left to right across a period, atomic radius usually decreases. This occurs because each successive element has an added proton and electron which causes the electron to be drawn closer to the nucleus.This decrease in atomic radius also causes the ionization energy to increase when moving from left to right across a period. The more tightly bound an element is, the more energy is required to remove an electron. Electronegativity increases in the same manner as ionization energy because of the pull exerted on the electrons by the nucleus. Electron affinity also shows a slight trend across a period. Metals (left side of a period) generally have a lower electron affinity than nonmetals (right side of a period) with the exception of the noble gases.
Blocks
Main article: Block (periodic table)
Because of the importance of the outermost electron shell, the different regions of the periodic table are sometimes referred to as periodic table blocks, named according to the subshell in which the "last" electron resides. The s-block comprises the first two groups (alkali metals and alkaline earth metals) as well as hydrogen and helium. The p-block comprises the last six groups which are groups 13 to 18 in IUPAC (3A to 8A in American) and contains, among others, all of the metalloids. The d-block comprises groups 3 to 12 in IUPAC (or 3B to 2B in American group numbering) and contains all of the transition metals. The f-block, usually offset below the rest of the periodic table, comprises the lanthanides and actinides.
Variations and other conventions
In presentations of the periodic table, the lanthanides and the actinides are customarily shown as two additional rows below the main body of the table, with placeholders or else a selected single element of each series (either lanthanum or lutetium, and either actinium or lawrencium, respectively) shown in a single cell of the main table, between barium and hafnium, and radium and rutherfordium, respectively. This convention is entirely a matter of aesthetics and formatting practicality; a rarely used wide-formatted periodic table inserts the lanthanide and actinide series in their proper places, as parts of the table's sixth and seventh rows (periods).

Many presentations of the periodic table show a dark stair-step diagonal line along the metalloids, with metals to the left of the line and non-metals to the right. Various other groupings of the chemical elements are sometimes also highlighted on a periodic table, such as transition metals, post-transition metals, and metalloids. Other informal groupings of the elements exist, such as the platinum group and the noble metals, but are rarely addressed in periodic tables.
Periodic trends
Main article: Periodic trends
The primary determinant of an element's chemical properties is its electron configuration, particularly the valence shell electrons. For instance, any atoms with four valence electrons occupying p orbitals will exhibit some similarity. The type of orbital in which the atom's outermost electrons reside determines the "block" to which it belongs. The number of valence shell electrons determines the family, or group, to which the element belongs. The total number of electron shells an atom has determines the period to which it belongs. Each shell is divided into different subshells, which as atomic number increases are filled roughly in the order depicted in the table at hand (according to the Aufbau principle; see table below). Hence the structure of the periodic table. Since the outermost electrons determine chemical properties, those with the same number of valence electrons are generally grouped together.
Subshell | s | f | d | p |
---|---|---|---|---|
Period | ||||
1 | 1s | |||
2 | 2s | 2p | ||
3 | 3s | 3p | ||
4 | 4s | 3d | 4p | |
5 | 5s | 4d | 5p | |
6 | 6s | 4f | 5d | 6p |
7 | 7s | 5f | 6d | 7p |
Progressing through a group from lightest element to heaviest element, the outer-shell electrons (those most readily accessible for participation in chemical reactions) are all in the same type of orbital, with a similar shape, but with increasingly higher energy and average distance from the nucleus. For instance, the outer-shell (or "valence") electrons of the first group, headed by hydrogen, all have one electron in an s orbital. In hydrogen, that s orbital is in the lowest possible energy state of any atom, the first-shell orbital (and represented by hydrogen's position in the first period of the table). In francium, the heaviest element of the group, the outer-shell electron is in the seventh-shell orbital, significantly further out on average from the nucleus than those electrons filling all the shells below it in energy. As another example, both carbon and lead have four electrons in their outer shell orbitals.
As atomic number (i.e., charge on the atomic nucleus) increases, the spin-orbit coupling between the nucleus and the electrons becomes greater, reducing the validity of the quantum mechanical orbital approximation model, which considers each atomic orbital as a separate entity.
Atomic and ionic radii
Main article: Atomic radius
Atomic radii vary in a predictable and explicable manner across the periodic table. For instance, the radii generally decrease along each period of the table, from the alkali metals to the noble gases; and increase down each group. The radius increases sharply between the noble gas at the end of each period and the alkali metal at the beginning of the next period. These trends of the atomic radii (and of various other chemical and physical properties of the elements) can be explained by the electron shell theory of the atom; they provided important evidence for the development and confirmation of quantum theory.
The way the atomic radius varies with increasing atomic number (Z) can be explained by the arrangement of electrons in shells of fixed capacity. The shells are generally filled in order of increasing radius, since the negatively charged electrons are attracted by the positively charged protons in the nucleus. As the atomic number increases along each row of the periodic table, the additional electrons go into the same outermost shell; whose radius gradually contracts, due to the increasing nuclear charge. In a noble gas, the outermost shell is completely filled; therefore, the additional electron of next alkali metal will go into the next outer shell, accounting for the sudden increase in the atomic radius.
The electrons in the 4f-subshell, which is progressively filled from cerium (Z = 58) to lutetium (Z = 71), are not particularly effective at shielding the increasing nuclear charge from the sub-shells further out. The elements immediately following the lanthanides have atomic radii which are smaller than would be expected and which are almost identical to the atomic radii of the elements immediately above them. Hence hafnium has virtually the same atomic radius (and chemistry) as zirconium, and tantalum has an atomic radius similar to niobium, and so forth. The effect of the lanthanide contraction is noticeable up to platinum (Z = 78), after which it is masked by a relativistic effect known as the inert pair effect.
The d-block contraction is less pronounced than the lanthanide contraction but arises from a similar cause. In this case, it is the poor shielding capacity of the 3d-electrons which affects the atomic radii and chemistries of the elements immediately following the first row of the transition metals, from gallium (Z = 31) to bromine (Z = 35).
Ionization energy and reactivity
Main articles: Ionization energy and Reactivity (chemistry)
The first ionization energy is the energy it takes to remove an electron from an atom, and the second ionization energy is the energy it takes to remove a second electron from an atom. Future ionization energies follow this same pattern. Higher ionization energies tend to be larger than lower ionization energies. Electrons in the closer orbitals experience greater forces of electrostatic attraction; thus, their removal requires increasingly more energy. Ionization energy becomes greater up and to the right of the periodic table.
Large jumps in the successive molar ionization energies occur when passing noble gas configurations. For example, as can be seen in the table above, the first two molar ionization energies of magnesium (stripping the two 3s electrons from a magnesium atom) are much smaller than the third, which requires stripping off a 2p electron from the very stable neon configuration of Mg.
Ionization energy is also a periodic trend within the periodic table organization. Moving left to right within a period or upward within a group, the first ionization energy generally increases. As the atomic radius decreases, it becomes harder to remove an electron that is closer to a more positively charged nucleus.
Electronegativity
Main article: Electronegativity
In general, electronegativity increases on passing from left to right along a period, and decreases on descending a group. Hence, fluorine is undoubtedly the most electronegative of the elements (not counting noble gases) while caesium is the least electronegative, at least of those elements for which substantial data is available.
There are some exceptions to this general rule. Gallium and germanium have higher electronegativities than aluminium and silicon respectively because of the d-block contraction. Elements of the fourth period immediately after the first row of the transition metals have unusually small atomic radii because the 3d-electrons are not effective at shielding the increased nuclear charge, and smaller atomic size correlates with higher electronegativity (see Allred-Rochow electronegativity, Sanderson electronegativity above). The anomalously high electronegativity of lead, particularly when compared to thallium and bismuth, appears to be an artifact of data selection (and data availability)—methods of calculation other than the Pauling method show the normal periodic trends for these elements.
Alternatives
Main article: Alternative periodic tables
While the iconic format presented above is widely used, other alternative periodic tables exist, including not only various rectangular formats, but also circular or cylindrical versions in which the rows (periods) flow from one into another, without the arbitrary breaks required at the margins of the usual printed or screen-formatted versions. Alternative periodic tables are developed often to highlight or emphasize different chemical or physical properties of the elements which are not as apparent in traditional periodic tables. Some tables aim to emphasize both the nucleon and electronic structure of atoms. This can be done by changing the spatial relationship or representation each element has with respect to another element in the table.
A common alternate layout is Charles Janet's Left Step Periodic Table, which organizes elements according to orbital filling. The modern version, known as the ADOMAH Periodic Table named after the biblical Adam, Adomah,, helps with writing electron configurations; the table is oriented 90˚ from the traditional periodic table, with the s-block moved to the end, after the noble gases.
Another alternative layout is Theodor Benfey's periodic table, where elements are arranged in a spiral with hydrogen at the center and spiraling outward, with the transition metals, lanthanides, and actinides as peninsulas.
Three-dimensional periodic tables exist as well, such as Paul-Antoine Giguère's periodic table, which has four billboards, each representing a block, with elements on the front and back. Hydrogen and helium are omitted.
Future developments and the end of the periodic table
Main article: Extended periodic tableAlthough all elements up to ununoctium have been discovered, only the first 108 and copernicium have known chemical properties. The other elements may behave differently from what would be predicted by extrapolation, due to relativistic effects; for example, flerovium has been predicted to possibly exhibit some noble-gas-like properties, even though it is currently placed in the carbon group.
It is unclear whether new elements will continue the pattern of the current periodic table as period 8, or require further adaptations or adjustments. Seaborg expected the eighth period, which includes a two-element s-block for elements 119 and 120, a g-block for the next 18 elements, and 30 additional elements continuing the current f-, d-, and p-blocks. On the other side, some physicists like Pekka Pyykkö have theorized that these additional elements do not follow the Madelung rule, which predicts how electron shells are filled, and thus affect the appearance of the present periodic table.
Richard Feynman noted that literally interpreting the relativistic Dirac equation has problems with electron orbitals at Z > 137, suggesting that neutral atoms cannot exist beyond untriseptium, and that a periodic table based on electron orbitals breaks down at this point. A more rigorous analysis calculates the limit to be Z ≈ 173.
Calculations from the Bohr model
The Bohr model has problems for atoms with atomic number greater than 137, because the speed of an electron in a 1s electron orbital, v, is given by
where Z is the atomic number, c is the speed of light, and α is the fine-structure constant. Under this model, any element with Z greater than 137 would require 1s electrons to be traveling faster than the speed of light. Thus, relativistic models must be used for Z > 137.
Calculations from the Dirac equation
The relativistic Dirac equation also has problems for Z > 137, for the ground state energy is
where m is the rest mass of the electron. Although for Z > 137, the wave function of the Dirac ground state is oscillatory, rather than bound, and there is no gap between the positive and negative energy spectra, as in the Klein paradox. More accurate calculations taking into account the effects of the finite size of the nucleus indicate that the binding energy first exceeds 2mc forZ > Zcr ≈ 173. For Z > Zcr, if the innermost orbital is not filled, the electric field of the nucleus will pull an electron out of the vacuum, resulting in the spontaneous emission of a positron.
See also
Template:Misplaced Pages books
- Abundance of the chemical elements
- Atomic electron configuration table
- Element collecting
- List of elements
- List of periodic table-related articles
- Table of nuclides
- Timeline of chemical elements discoveries
References
- Siegfried, Robert (2002). From elements to atoms: a history of chemical composition. Philadelphia, Pennsylvania: Library of Congress Cataloging-in-Publication Data. p. 92. ISBN 0-87169-924-9.
{{cite book}}
:|access-date=
requires|url=
(help) - ^ Ball, p. 100
- Horvitz, Leslie (2002). Eureka!: Scientific Breakthroughs That Changed The World. New York: John Wiley. p. 43. ISBN 978-0-471-23341-1. OCLC 50766822.
- Ball, p. 101
- Newlands, John A. R. (20 August 1864). "On Relations Among the Equivalents". Chemical News. 10: 94–95.
- Newlands, John A. R. (18 August 1865). "On the Law of Octaves". Chemical News. 12: 83.
- Bryson, Bill (2004). A Short History of Nearly Everything. Black Swan. pp. 141–142. ISBN 978-0-552-15174-0.
- Brock, W. H.; Knight (1965). "The Atomic Debates: 'Memorable and Interesting Evenings in the Life of the Chemical Society'". Isis. 56 (1). The University of Chicago Press: 5–25.
{{cite journal}}
:|first3=
missing|last3=
(help) - Mendelejew, Dimitri (1869). "Über die Beziehungen der Eigenschaften zu den Atomgewichten der Elemente". Zeitschrift für Chemie (in German): 405–406.
- Ball, pp. 100–102
- Pullman, Bernard (1998). The Atom in the History of Human Thought. Translated by Axel Reisinger. Oxford University Press. p. 227. ISBN 0-19-515040-6.
- Ball, p. 105
- Atkins, P. W. (1995). The Periodic Kingdom. HarperCollins Publishers, Inc. p. 87. ISBN 0-465-07265-8.
- Kaji, Masanori (2002). "D.I. Mendeleev's Concept of Chemical Elements and the Principle of Chemistry" (PDF). Bull. Hist. Chem. 27 (1). Tokyo Institute of Technology: 4–16. Retrieved 11 June 2012.
- Adloff, Jean-Pierre; Kaufman, George B. (25 September 2005). "Francium (Atomic Number 87), the Last Discovered Natural Element". The Chemical Educator. Retrieved 26 March 2007.
- Hoffman, D. C.; Lawrence, F. O.; Mewherter, J. L.; Rourke, F. M. (1971). "Detection of Plutonium-244 in Nature". Nature. 234 (5325): 132–134. Bibcode:1971Natur.234..132H. doi:10.1038/234132a0.
- ^ Emsley, John (2011). Nature's Building Blocks: An A-Z Guide to the Elements (New ed.). New York, NY: Oxford University Press. ISBN 978-0-19-960563-7.
- Ball, p. 111
- Fluck, E. (1988). "New Notations in the Periodic Table" (PDF). Pure Appl. Chem. 60 (3). IUPAC: 431–436. doi:10.1351/pac198860030431. Retrieved 24 March 2012.
- Ball, p. 123
- Barber, Robert C.; Karol, Paul J; Nakahara, Hiromichi; Vardaci, Emanuele; Vogt, Erich W. (2011). "Discovery of the elements with atomic numbers greater than or equal to 113 (IUPAC Technical Report)". Pure Appl. Chem. 83 (7): 1485. doi:10.1351/PAC-REP-10-05-01.
{{cite journal}}
: CS1 maint: multiple names: authors list (link) - Template:Ru icon "Эксперимент по синтезу 117-го элемента получает продолжение". JINR. 2012.
{{cite web}}
: Unknown parameter|trans_title=
ignored (|trans-title=
suggested) (help) - Meija, Juris; et al. (2016). "Atomic weights of the elements 2013 (IUPAC Technical Report)". Pure and Applied Chemistry. 88 (3): 265–291. doi:10.1515/pac-2015-0305.
- Prohaska, Thomas; Irrgeher, Johanna; Benefield, Jacqueline; Böhlke, John K.; Chesson, Lesley A.; Coplen, Tyler B.; Ding, Tiping; Dunn, Philip J. H.; Gröning, Manfred; Holden, Norman E.; Meijer, Harro A. J. (4 May 2022). "Standard atomic weights of the elements 2021 (IUPAC Technical Report)". Pure and Applied Chemistry. doi:10.1515/pac-2019-0603. ISSN 1365-3075.
- Gray, p. 6
- Koppenol, W. H. (2002). "Naming of New Elements (IUPAC Recommendations 2002)" (PDF). Pure and Applied Chemistry. 74 (5): 787–791. doi:10.1351/pac200274050787.
- Haire, Richard G. (2006). "Fermium, Mendelevium, Nobelium and Lawrencium". In Morss; Edelstein, Norman M.; Fuger, Jean (eds.). The Chemistry of the Actinide and Transactinide Elements (3rd ed.). Dordrecht, The Netherlands: Springer Science+Business Media. ISBN 1-4020-3555-1.
{{cite book}}
: CS1 maint: ref duplicates default (link) - Gray, p. 233
- Kaplan SAT Subject Test: Chemistry 2009–2012 Edition. Kaplan Publishing. 2009. ISBN 978-1-4195-5260-1.
- ^ Greenwood, Norman N.; Earnshaw, Alan (1984). Chemistry of the Elements. Oxford: Pergamon Press. pp. 24–27. ISBN 978-0-08-022057-4.
- ^ Cronyn, Marshall W. (2003). "The Proper Place for Hydrogen in the Periodic Table". Journal of Chemical Education. 80 (8): 947–951. Bibcode:2003JChEd..80..947C. doi:10.1021/ed080p947.
{{cite journal}}
: Unknown parameter|month=
ignored (help) - ^ Gray, p. 12
- Samanta, C.; Chowdhury, P. Roy; Basu, D.N. (2007). "Predictions of alpha decay half lives of heavy and superheavy elements". Nucl. Phys. A. 789: 142–154. arXiv:nucl-th/0703086. Bibcode:2007NuPhA.789..142S. doi:10.1016/j.nuclphysa.2007.04.001.
- ^ Leigh, G. J. (1990). Nomenclature of Inorganic Chemistry: Recommendations 1990. Blackwell Science. ISBN 0-632-02494-1.
- Leigh, Jeffery. "Periodic Tables and IUPAC". Chemistry International: The News Magazine of The International Union of Pure and Applied Chemistry (IUPAC). Retrieved 23 March 2011.
- Scerri, E. R. (2007). The Periodic Table: Its Story and its Significance. Oxford University Press. ISBN 978-0-19-530573-9.
- ^ Moore, p. 111
- Stoker, Stephen H. (2007). General, organic, and biological chemistry. New York: Houghton Mifflin. p. 68. ISBN 978-0-618-73063-6. OCLC 52445586.
- Mascetta, Joseph (2003). Chemistry The Easy Way (4th ed.). New York: Hauppauge. p. 50. ISBN 978-0-7641-1978-1. OCLC 52047235.
- Kotz, John; Treichel, Paul; Townsend, John (2009). Chemistry and Chemical Reactivity, Volume 2 (7th ed.). Belmont: Thomson Brooks/Cole. p. 324. ISBN 978-0-495-38712-1. OCLC 220756597.
- Jones, Chris (2002). d- and f-block chemistry. New York: J. Wiley & Sons. p. 2. ISBN 978-0-471-22476-1. OCLC 300468713.
- Gray, p. 11
- ^ Attention: This template ({{cite doi}}) is deprecated. To cite the publication identified by doi:10.1021/ed064p9, please use {{cite journal}} (if it was published in a bona fide academic journal, otherwise {{cite report}} with
|doi=10.1021/ed064p9
instead. - Moore, p. 46
- Attention: This template ({{cite doi}}) is deprecated. To cite the publication identified by doi:10.1063/1.432900, please use {{cite journal}} (if it was published in a bona fide academic journal, otherwise {{cite report}} with
|doi=10.1063/1.432900
instead. - Hornback, Joseph (2006). Organic Chemistry (2nd ed.). Pacific Grove: Thomson Brooks/Cole. p. 62. ISBN 978-0-534-49317-2. OCLC 66441248.
- Gray, pp. 25, 189
- Bethe, Hans; Salpeter, E. E. (1957). "Quantum Mechanics of One- and Two-Electron Atoms" (PDF). Encyclopedia of Physics. 35: 347–385. Retrieved 11 April 2012.
- ^ Greenwood, Norman N.; Earnshaw, Alan (1984). Chemistry of the Elements. Oxford: Pergamon Press. p. 27. ISBN 978-0-08-022057-4.
- ^ Jolly, W. L. (1991). Modern Inorganic Chemistry (2nd ed.). McGraw-Hill. p. 22. ISBN 978-0-07-112651-9.
- ^ Greenwood, Norman N.; Earnshaw, Alan (1984). Chemistry of the Elements. Oxford: Pergamon Press. p. 28. ISBN 978-0-08-022057-4.
- ^ Greenwood, Norman N.; Earnshaw, Alan (1984). Chemistry of the Elements. Oxford: Pergamon Press. p. 30. ISBN 978-0-08-022057-4.
- Allred, A. L. (1960). "Electronegativity values from thermochemical data". Journal of Inorganic and Nuclear Chemistry. 17 (3–4). Northwestern University: 215–221. doi:10.1016/0022-1902(61)80142-5. Retrieved 11 June 2012.
- Tsimmerman, Valery (19 March 2009). "Periodic Law can be understood in terms of the Tetrahedral Sphere Packing!". GoDaddy.com. Retrieved 5 July 2012.
- Stewart, Philip J. (2009). "Charles Janet: unrecognized genius of the periodic system". Foundations of Chemistry. 12 (1). doi:10.1007/s10698-008-9062-5.
{{cite journal}}
:|access-date=
requires|url=
(help); Check date values in:|accessdate=
(help) - Seaborg, Glenn (1964). "Plutonium: The Ornery Element". Chemistry. 37 (6): 14.
- Mazurs, E.G. (1974). Graphical Representations of the Periodic System During One Hundred Years. Alabama: University of Alabama Press. ISBN 9780817332006.
{{cite book}}
:|access-date=
requires|url=
(help) - Schändel, Matthias (2003). The Chemistry of Superheavy Elements. Dordrecht: Kluwer Academic Publishers. p. 277. ISBN 1-4020-1250-0.
{{cite book}}
:|access-date=
requires|url=
(help) - Frazier, K. (1978). "Superheavy Elements". Science News. 113 (15): 236–238. doi:10.2307/3963006. JSTOR 3963006.
- Pyykkö, Pekka (2011). "A suggested periodic table up to Z ≤ 172, based on Dirac–Fock calculations on atoms and ions". Physical Chemistry Chemical Physics. 13 (1): 161–8. Bibcode:2011PCCP...13..161P. doi:10.1039/c0cp01575j. PMID 20967377.
- Elert, G. "Atomic Models". The Physics Hypertextbook. Retrieved 9 October 2009.
- Greiner, W.; Schramm, S. (2008). "American Journal of Physics". 76: 509.
{{cite journal}}
: Cite journal requires|journal=
(help) - Eisberg, R.; Resnick, R. (1974). Quantum Physics of Atoms, Molecules, Solids, Nuclei and Particles. John Wiley & Sons. ISBN 9780471873730.
-
Bjorken, J. D.; Drell, S. D. (1965). Relativistic Quantum Mechanics. American Institute of Physics. ISBN 0031-9228.
{{cite book}}
: Check|isbn=
value: length (help) -
Attention: This template ({{cite doi}}) is deprecated. To cite the publication identified by doi:10.1016/j.physrep.2009.10.004, please use {{cite journal}} (if it was published in a bona fide academic journal, otherwise {{cite report}} with
|doi=10.1016/j.physrep.2009.10.004
instead.
Bibliography
- Ball, Philip (2002). The Ingredients: A Guided Tour of the Elements. Oxford University Press. ISBN 0-19-284100-9.
- Bouma, J. (1989). "An Application-Oriented Periodic Table of the Elements". J. Chem. Ed. 66 (9): 741. Bibcode:1989JChEd..66..741B. doi:10.1021/ed066p741.
- Gray, Theodore (2009). The Elements: A Visual Exploration of Every Known Atom in the Universe. New York: Black Dog & Leventhal Publishers. ISBN 978-1-57912-814-2.
- Hjørland, Birger (2011). "The periodic table and the philosophy of classification". Knowledge Organization. 38 (1): 9–21. Retrieved 13 March 2011.
- Kean, Sam (2010). The Disappearing Spoon - and other true tales from the Periodic Table. London: Black Swan. ISBN 978-0-552-77750-6.
- Levi, Primo (1984). The Periodic Table . London: Penguin Books. ISBN 978-0-14-139944-7.
- Mazurs, E.G (1974). Graphical Representations of the Periodic System During One Hundred Years. Alabama: University of Alabama Press.
- Moore, John (2003). Chemistry For Dummies. New York: Wiley Publications. p. 111. ISBN 978-0-7645-5430-8. OCLC 51168057.
- Scerri, Eric (2007). The periodic table: its story and its significance. Oxford: Oxford University Press. ISBN 0-19-530573-6.
External links
- M. Dayah. "Dynamic Periodic Table". Retrieved 14 May 2012.
- Brady Haran. "The Periodic Table of Videos". University of Nottingham. Retrieved 14 May 2012.
- Mark Winter. "WebElements: the periodic table on the web". University of Sheffield. Retrieved 14 May 2012.
- Mark R. Leach. "The INTERNET Database of Periodic Tables". Retrieved 14 May 2012.
- "Periodic Table of the Elements in Four Hundred Languages". Retrieved 14 May 2012.
Periodic table | |||||||||||||||||||||||||||||||||||||||||||||||||||||||||||||||||||||||||||||||||||||||||||||||||||||||||||||||||||||||||||||||||||||||||||||||||||||||||||||||||||||||||||||||||||||||||||||||||||||||||||||||||||||||||||||||||||||||||||||||||||||||||||||||||||||||||
---|---|---|---|---|---|---|---|---|---|---|---|---|---|---|---|---|---|---|---|---|---|---|---|---|---|---|---|---|---|---|---|---|---|---|---|---|---|---|---|---|---|---|---|---|---|---|---|---|---|---|---|---|---|---|---|---|---|---|---|---|---|---|---|---|---|---|---|---|---|---|---|---|---|---|---|---|---|---|---|---|---|---|---|---|---|---|---|---|---|---|---|---|---|---|---|---|---|---|---|---|---|---|---|---|---|---|---|---|---|---|---|---|---|---|---|---|---|---|---|---|---|---|---|---|---|---|---|---|---|---|---|---|---|---|---|---|---|---|---|---|---|---|---|---|---|---|---|---|---|---|---|---|---|---|---|---|---|---|---|---|---|---|---|---|---|---|---|---|---|---|---|---|---|---|---|---|---|---|---|---|---|---|---|---|---|---|---|---|---|---|---|---|---|---|---|---|---|---|---|---|---|---|---|---|---|---|---|---|---|---|---|---|---|---|---|---|---|---|---|---|---|---|---|---|---|---|---|---|---|---|---|---|---|---|---|---|---|---|---|---|---|---|---|---|---|---|---|---|---|---|---|---|---|---|---|---|---|---|---|---|---|---|---|---|---|
| |||||||||||||||||||||||||||||||||||||||||||||||||||||||||||||||||||||||||||||||||||||||||||||||||||||||||||||||||||||||||||||||||||||||||||||||||||||||||||||||||||||||||||||||||||||||||||||||||||||||||||||||||||||||||||||||||||||||||||||||||||||||||||||||||||||||||
|
Periodic table | |||||||||||||||||||
---|---|---|---|---|---|---|---|---|---|---|---|---|---|---|---|---|---|---|---|
Periodic table forms | |||||||||||||||||||
Sets of elements |
| ||||||||||||||||||
Elements | |||||||||||||||||||
History | |||||||||||||||||||
See also | |||||||||||||||||||
Template:Link FL Template:Link GA
Categories: