Cambrian | ||||||||||||||||||||||||||||||||||||||
---|---|---|---|---|---|---|---|---|---|---|---|---|---|---|---|---|---|---|---|---|---|---|---|---|---|---|---|---|---|---|---|---|---|---|---|---|---|---|
538.8 ± 0.6 – 486.85 ± 1.5 Ma PreꞒ Ꞓ O S D C P T J K Pg N | ||||||||||||||||||||||||||||||||||||||
![]() | ||||||||||||||||||||||||||||||||||||||
Chronology | ||||||||||||||||||||||||||||||||||||||
| ||||||||||||||||||||||||||||||||||||||
Etymology | ||||||||||||||||||||||||||||||||||||||
Name formality | Formal | |||||||||||||||||||||||||||||||||||||
Usage information | ||||||||||||||||||||||||||||||||||||||
Celestial body | Earth | |||||||||||||||||||||||||||||||||||||
Regional usage | Global (ICS) | |||||||||||||||||||||||||||||||||||||
Time scale(s) used | ICS Time Scale | |||||||||||||||||||||||||||||||||||||
Definition | ||||||||||||||||||||||||||||||||||||||
Chronological unit | Period | |||||||||||||||||||||||||||||||||||||
Stratigraphic unit | System | |||||||||||||||||||||||||||||||||||||
First proposed by | Adam Sedgwick, 1835 | |||||||||||||||||||||||||||||||||||||
Time span formality | Formal | |||||||||||||||||||||||||||||||||||||
Lower boundary definition | Appearance of the Ichnofossil Treptichnus pedum | |||||||||||||||||||||||||||||||||||||
Lower boundary GSSP | Fortune Head section, Newfoundland, Canada 47°04′34″N 55°49′52″W / 47.0762°N 55.8310°W / 47.0762; -55.8310 | |||||||||||||||||||||||||||||||||||||
Lower GSSP ratified | 1992 | |||||||||||||||||||||||||||||||||||||
Upper boundary definition | FAD of the Conodont Iapetognathus fluctivagus. | |||||||||||||||||||||||||||||||||||||
Upper boundary GSSP | Greenpoint section, Green Point, Newfoundland, Canada 49°40′58″N 57°57′55″W / 49.6829°N 57.9653°W / 49.6829; -57.9653 | |||||||||||||||||||||||||||||||||||||
Upper GSSP ratified | 2000 | |||||||||||||||||||||||||||||||||||||
Atmospheric and climatic data | ||||||||||||||||||||||||||||||||||||||
Sea level above present day | Rising steadily from 4 m to 90 m |
Part of a series on |
The Cambrian explosion |
---|
![]() |
Fossil localities |
Key organisms |
Evolutionary concepts
|
The Cambrian ( /ˈkæmbri.ən, ˈkeɪm-/ KAM-bree-ən, KAYM-) is the first geological period of the Paleozoic Era, and the Phanerozoic Eon. The Cambrian lasted 51.95 million years from the end of the preceding Ediacaran period 538.8 Ma (million years ago) to the beginning of the Ordovician Period 486.85 Ma.
Most of the continents lay in the southern hemisphere surrounded by the vast Panthalassa Ocean. The assembly of Gondwana during the Ediacaran and early Cambrian led to the development of new convergent plate boundaries and continental-margin arc magmatism along its margins that helped drive up global temperatures. Laurentia lay across the equator, separated from Gondwana by the opening Iapetus Ocean.
The Cambrian was a time of greenhouse climate conditions, with high levels of atmospheric carbon dioxide and low levels of oxygen in the atmosphere and seas. Upwellings of anoxic deep ocean waters into shallow marine environments led to extinction events, whilst periods of raised oxygenation led to increased biodiversity.
The Cambrian marked a profound change in life on Earth; prior to the Period, the majority of living organisms were small, unicellular and poorly preserved. Complex, multicellular organisms gradually became more common during the Ediacaran, but it was not until the Cambrian that the rapid diversification of lifeforms, known as the Cambrian explosion, produced the first representatives of most modern animal phyla. The Period is also unique in its unusually high proportion of lagerstätte deposits, sites of exceptional preservation where "soft" parts of organisms are preserved as well as their more resistant shells.
By the end of the Cambrian, myriapods, arachnids, and hexapods started adapting to the land, along with the first plants.
Etymology and history
The term Cambrian is derived from the Latin version of Cymru, the Welsh name for Wales, where rocks of this age were first studied. It was named by Adam Sedgwick in 1835, who divided it into three groups; the Lower, Middle, and Upper. He defined the boundary between the Cambrian and the overlying Silurian, together with Roderick Murchison, in their joint paper "On the Silurian and Cambrian Systems, Exhibiting the Order in which the Older Sedimentary Strata Succeed each other in England and Wales". This early agreement did not last.
Due to the scarcity of fossils, Sedgwick used rock types to identify Cambrian strata. He was also slow in publishing further work. The clear fossil record of the Silurian, however, allowed Murchison to correlate rocks of a similar age across Europe and Russia, and on these he published extensively. As increasing numbers of fossils were identified in older rocks, he extended the base of the Silurian downwards into the Sedgwick's "Upper Cambrian", claiming all fossilised strata for "his" Silurian series. Matters were complicated further when, in 1852, fieldwork carried out by Sedgwick and others revealed an unconformity within the Silurian, with a clear difference in fauna between the two. This allowed Sedgwick to now claim a large section of the Silurian for "his" Cambrian and gave the Cambrian an identifiable fossil record. The dispute between the two geologists and their supporters, over the boundary between the Cambrian and Silurian, would extend beyond the life times of both Sedgwick and Murchison. It was not resolved until 1879, when Charles Lapworth proposed the disputed strata belong to its own system, which he named the Ordovician.
The term Cambrian for the oldest period of the Paleozoic was officially agreed in 1960, at the 21st International Geological Congress. It only includes Sedgwick's "Lower Cambrian series", but its base has been extended into much older rocks.
Geology
Stratigraphy
Systems, series and stages can be defined globally or regionally. For global stratigraphic correlation, the ICS ratify rock units based on a Global Boundary Stratotype Section and Point (GSSP) from a single formation (a stratotype) identifying the lower boundary of the unit. Currently the boundaries of the Cambrian System, three series and six stages are defined by global stratotype sections and points.
Ediacaran-Cambrian boundary
The lower boundary of the Cambrian was originally held to represent the first appearance of complex life, represented by trilobites. The recognition of small shelly fossils before the first trilobites, and Ediacara biota substantially earlier, has led to calls for a more precisely defined base to the Cambrian Period.
Despite the long recognition of its distinction from younger Ordovician rocks and older Precambrian rocks, it was not until 1994 that the Cambrian system/period was internationally ratified. After decades of careful consideration, a continuous sedimentary sequence at Fortune Head, Newfoundland was settled upon as a formal base of the Cambrian Period, which was to be correlated worldwide by the earliest appearance of Treptichnus pedum. Discovery of this fossil a few metres below the GSSP led to the refinement of this statement, and it is the T. pedum ichnofossil assemblage that is now formally used to correlate the base of the Cambrian.
This formal designation allowed radiometric dates to be obtained from samples across the globe that corresponded to the base of the Cambrian. An early date of 570 Ma quickly gained favour, though the methods used to obtain this number are now considered to be unsuitable and inaccurate. A more precise analysis using modern radiometric dating yields a date of 538.8 ± 0.6 Ma. The ash horizon in Oman from which this date was recovered corresponds to a marked fall in the abundance of carbon-13 that correlates to equivalent excursions elsewhere in the world, and to the disappearance of distinctive Ediacaran fossils (Namacalathus, Cloudina). Nevertheless, there are arguments that the dated horizon in Oman does not correspond to the Ediacaran-Cambrian boundary, but represents a facies change from marine to evaporite-dominated strata – which would mean that dates from other sections, ranging from 544 to 542 Ma, are more suitable.
International Series | International Stage | Chinese | Australian | Russian-Kazakhian | North American | European | |
---|---|---|---|---|---|---|---|
C a m b r i a n |
Furongian | "Stage 10" | Niuchehean | Datsonian | Batyrbaian | Skullrockian / Ibexian (part) | Merionethian |
Payntonian | Sunwaptan / Trempealeauan | ||||||
Jiangshanian | Jiangshanian | Iverian | Aksaian | ||||
Sakian | |||||||
Paibian | Paibian | Idamean | Steptoean / Franconian | ||||
Miaolingian | Guzhangian | Guzhangian | Mindyallan | Ayusokkanian | Marjuman / Dresbachian | ||
Boomerangian | Mayan | Acadian / St. David's | |||||
Drumian | Wangcunian | Undillian | |||||
Florian | |||||||
Wuliuan | Wuliuan | Templetonian | Amgan / Amgaian | Topazan | |||
Ordian | Delmaran | ||||||
Cambrian Series 2 | "Stage 4" | Duyunian | Branchian / Comley (part) | ||||
Toyonian | |||||||
Dyeran | |||||||
Botomian | |||||||
"Stage 3" | Nangaoan | ||||||
Atdabanian | Montezuman | ||||||
Placentian / Comley (part) | |||||||
Terreneuvian | "Stage 2" | Meishucunian | Tommotian* | Begadean | |||
Jinningian | Nemakit-Daldynian* | ||||||
Fortunian | |||||||
Ediacaran | Sinian | Adelaidean | Sakharan / Vendian | Hadrynian |
*Most Russian paleontologists define the lower boundary of the Cambrian at the base of the Tommotian Stage, characterized by diversification and global distribution of organisms with mineral skeletons and the appearance of the first Archaeocyath bioherms.
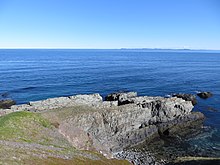
Terreneuvian
The Terreneuvian is the lowermost series/epoch of the Cambrian, lasting from 538.8 ± 0.6 Ma to c. 521 Ma. It is divided into two stages: the Fortunian stage, 538.8 ± 0.6 Ma to c. 529 Ma; and the unnamed Stage 2, c. 529 Ma to c. 521 Ma. The name Terreneuvian was ratified by the International Union of Geological Sciences (IUGS) in 2007, replacing the previous "Cambrian Series 1". The GSSP defining its base is at Fortune Head on the Burin Peninsula, eastern Newfoundland, Canada (see Ediacaran - Cambrian boundary above). The Terreneuvian is the only series in the Cambrian to contain no trilobite fossils. Its lower part is characterised by complex, sediment-penetrating Phanerozoic-type trace fossils, and its upper part by small shelly fossils.
Cambrian Series 2
The second series/epoch of the Cambrian is currently unnamed and known as Cambrian Series 2. It lasted from c. 521 Ma to c. 506.5 Ma. Its two stages are also unnamed and known as Cambrian Stage 3, c. 521 Ma to c. 514.5 Ma, and Cambrian Stage 4, c. 514.5 Ma to c. 506.5 Ma. The base of Series 2 does not yet have a GSSP, but it is expected to be defined in strata marking the first appearance of trilobites in Gondwana. There was a rapid diversification of metazoans during this epoch, but their restricted geographic distribution, particularly of the trilobites and archaeocyaths, have made global correlations difficult, hence ongoing efforts to establish a GSSP.
Miaolingian

The Miaolingian is the third series/epoch of the Cambrian, lasting from c. 506.5 Ma to c. 497 Ma, and roughly identical to the middle Cambrian in older literature . It is divided into three stages: the Wuliuan c. 506.5 Ma to 504.5 Ma; the Drumian c. 504.5 Ma to c. 500.5 Ma; and the Guzhangian c. 500.5 Ma to c. 497 Ma. The name replaces Cambrian Series 3 and was ratified by the IUGS in 2018. It is named after the Miaoling Mountains in southeastern Guizhou Province, South China, where the GSSP marking its base is found. This is defined by the first appearance of the oryctocephalid trilobite Oryctocephalus indicus. Secondary markers for the base of the Miaolingian include the appearance of many acritarchs forms, a global marine transgression, and the disappearance of the polymerid trilobites, Bathynotus or Ovatoryctocara. Unlike the Terreneuvian and Series 2, all the stages of the Miaolingian are defined by GSSPs.
The olenellids, eodiscids, and most redlichiids trilobites went extinct at the boundary between Series 2 and the Miaolingian. This is considered the oldest mass extinction of trilobites.
Furongian
The Furongian, c. 497 Ma to 486.85 ± 1.5 Ma, is the fourth and uppermost series/epoch of the Cambrian. The name was ratified by the IUGS in 2003 and replaces Cambrian Series 4 and the traditional "Upper Cambrian". The GSSP for the base of the Furongian is in the Wuling Mountains, in northwestern Hunan Province, China. It coincides with the first appearance of the agnostoid trilobite Glyptagnostus reticulatus, and is near the beginning of a large positive δC isotopic excursion.
The Furongian is divided into three stages: the Paibian, c. 497 Ma to c. 494 Ma, and the Jiangshanian c. 494.2 Ma to c. 491 Ma, which have defined GSSPs; and the unnamed Cambrian Stage 10, c. 491 Ma to 486.85 ± 1.5 Ma.
Cambrian–Ordovician boundary
The GSSP for the Cambrian–Ordovician boundary is at Green Point, western Newfoundland, Canada, and is dated at 486.85 Ma. It is defined by the appearance of the conodont Iapetognathus fluctivagus. Where these conodonts are not found the appearance of planktonic graptolites or the trilobite Jujuyaspis borealis can be used. The boundary also corresponds with the peak of the largest positive variation in the δC curve during the boundary time interval and with a global marine transgression.
Impact structures
Major meteorite impact structures include: the early Cambrian (c. 535 Ma) Neugrund crater in the Gulf of Finland, Estonia, a complex meteorite crater about 20 km in diameter, with two inner ridges of about 7 km and 6 km diameter, and an outer ridge of 8 km that formed as the result of an impact of an asteroid 1 km in diameter; the 5 km diameter Gardnos crater (500±10 Ma) in Buskerud, Norway, where post-impact sediments indicate the impact occurred in a shallow marine environment with rock avalanches and debris flows occurring as the crater rim was breached not long after impact; the 24 km diameter Presqu'ile crater (500 Ma or younger) Quebec, Canada; the 19 km diameter Glikson crater (c. 508 Ma) in Western Australia; the 5 km diameter Mizarai crater (500±10 Ma) in Lithuania; and the 3.2 km diameter Newporte structure (c. 500 Ma or slightly younger) in North Dakota, U.S.A.
Paleogeography
Reconstructing the position of the continents during the Cambrian is based on palaeomagnetic, palaeobiogeographic, tectonic, geological and palaeoclimatic data. However, these have different levels of uncertainty and can produce contradictory locations for the major continents. This, together with the ongoing debate around the existence of the Neoproterozoic supercontinent of Pannotia, means that while most models agree the continents lay in the southern hemisphere, with the vast Panthalassa Ocean covering most of northern hemisphere, the exact distribution and timing of the movements of the Cambrian continents varies between models.
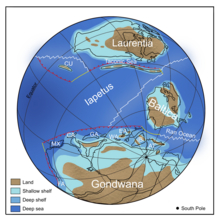
Most models show Gondwana stretching from the south polar region to north of the equator. Early in the Cambrian, the south pole corresponded with the western South American sector and as Gondwana rotated anti-clockwise, by the middle of the Cambrian, the south pole lay in the northwest African region.
Laurentia lay across the equator, separated from Gondwana by the Iapetus Ocean. Proponents of Pannotia have Laurentia and Baltica close to the Amazonia region of Gondwana with a narrow Iapetus Ocean that only began to open once Gondwana was fully assembled c. 520 Ma. Those not in favour of the existence of Pannotia show the Iapetus opening during the Late Neoproterozoic, with up to c. 6,500 km (c. 4038 miles) between Laurentia and West Gondwana at the beginning of the Cambrian.
Of the smaller continents, Baltica lay between Laurentia and Gondwana, the Ran Ocean (an arm of the Iapetus) opening between it and Gondwana. Siberia lay close to the western margin of Gondwana and to the north of Baltica. Annamia and South China formed a single continent situated off north central Gondwana. The location of North China is unclear. It may have lain along the northeast Indian sector of Gondwana or already have been a separate continent.
Laurentia
During the Cambrian, Laurentia lay across or close to the equator. It drifted south and rotated c. 20° anticlockwise during the middle Cambrian, before drifting north again in the late Cambrian.
After the Late Neoproterozoic (or mid-Cambrian) rifting of Laurentia from Gondwana and the subsequent opening of the Iapetus Ocean, Laurentia was largely surrounded by passive margins with much of the continent covered by shallow seas.
As Laurentia separated from Gondwana, a sliver of continental terrane rifted from Laurentia with the narrow Taconic seaway opening between them. The remains of this terrane are now found in southern Scotland, Ireland, and Newfoundland. Intra-oceanic subduction either to the southeast of this terrane in the Iapetus, or to its northwest in the Taconic seaway, resulted in the formation of an island arc. This accreted to the terrane in the late Cambrian, triggering southeast-dipping subduction beneath the terrane itself and consequent closure of the marginal seaway. The terrane collided with Laurentia in the Early Ordovician.
Towards the end of the early Cambrian, rifting along Laurentia's southeastern margin led to the separation of Cuyania (now part of Argentina) from the Ouachita embayment with a new ocean established that continued to widen through the Cambrian and Early Ordovician.
Gondwana
Gondwana was a massive continent, three times the size of any of the other Cambrian continents. Its continental land area extended from the south pole to north of the equator. Around it were extensive shallow seas and numerous smaller land areas.
The cratons that formed Gondwana came together during the Neoproterozoic to early Cambrian. A narrow ocean separated Amazonia from Gondwana until c. 530 Ma and the Arequipa-Antofalla block united with the South American sector of Gondwana in the early Cambrian. The Kuunga Orogeny between northern (Congo Craton, Madagascar and India) and southern Gondwana (Kalahari Craton and East Antarctica), which began c. 570 Ma, continued with parts of northern Gondwana over-riding southern Gondwana and was accompanied by metamorphism and the intrusion of granites.
Subduction zones, active since the Neoproterozoic, extended around much of Gondwana's margins, from northwest Africa southwards round South America, South Africa, East Antarctica, and the eastern edge of West Australia. Shorter subduction zones existed north of Arabia and India.
The Famatinian continental arc stretched from central Peru in the north to central Argentina in the south. Subduction beneath this proto-Andean margin began by the late Cambrian.
Along the northern margin of Gondwana, between northern Africa and the Armorican Terranes of southern Europe, the continental arc of the Cadomian Orogeny continued from the Neoproterozoic in response to the oblique subduction of the Iapetus Ocean. This subduction extended west along the Gondwanan margin and by c. 530 Ma may have evolved into a major transform fault system.
At c. 511 Ma the continental flood basalts of the Kalkarindji large igneous province (LIP) began to erupt. These covered an area of > 2.1 × 10 km across northern, central and Western Australia regions of Gondwana making it one of the largest, as well as the earliest, LIPs of the Phanerozoic. The timing of the eruptions suggests they played a role in the early to middle Cambrian mass extinction.
Ganderia, East and West Avalonia, Carolinia and Meguma Terranes
The terranes of Ganderia, East and West Avalonia, Carolinia and Meguma lay in polar regions during the early Cambrian, and high-to-mid southern latitudes by the mid to late Cambrian. They are commonly shown as an island arc-transform fault system along the northwestern margin of Gondwana north of northwest Africa and Amazonia, which rifted from Gondwana during the Ordovician. However, some models show these terranes as part of a single independent microcontinent, Greater Avalonia, lying to the west of Baltica and aligned with its eastern (Timanide) margin, with the Iapetus to the north and the Ran Ocean to the south.
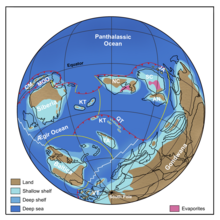
Baltica
During the Cambrian, Baltica rotated more than 60° anti-clockwise and began to drift northwards. This rotation was accommodated by major strike-slip movements in the Ran Ocean between it and Gondwana.
Baltica lay at mid-to-high southerly latitudes, separated from Laurentia by the Iapetus and from Gondwana by the Ran Ocean. It was composed of two continents, Fennoscandia and Sarmatia, separated by shallow seas. The sediments deposited in these unconformably overlay Precambrian basement rocks. The lack of coarse-grained sediments indicates low lying topography across the centre of the craton.
Along Baltica's northeastern margin subduction and arc magmatism associated with the Ediacaran Timanian Orogeny was coming to an end. In this region the early to middle Cambrian was a time of non-deposition and followed by late Cambrian rifting and sedimentation.
Its southeastern margin was also a convergent boundary, with the accretion of island arcs and microcontinents to the craton, although the details are unclear.
Siberia
Siberia began the Cambrian close to western Gondwana and north of Baltica. It drifted northwestwards to close to the equator as the Ægir Ocean opened between it and Baltica. Much of the continent was covered by shallow seas with extensive archaeocyathan reefs. The then northern third of the continent (present day south; Siberia has rotated 180° since the Cambrian) adjacent to its convergent margin was mountainous.
From the Late Neoproterozoic to the Ordovician, a series of island arcs accreted to Siberia's then northeastern margin, accompanied by extensive arc and back-arc volcanism. These now form the Altai-Sayan terranes. Some models show a convergent plate margin extending from Greater Avalonia, through the Timanide margin of Baltica, forming the Kipchak island arc offshore of southeastern Siberia and curving round to become part of the Altai-Sayan convergent margin.
Along the then western margin, Late Neoproterozoic to early Cambrian rifting was followed by the development of a passive margin.
To the then north, Siberia was separated from the Central Mongolian terrane by the narrow and slowly opening Mongol-Okhotsk Ocean. The Central Mongolian terrane's northern margin with the Panthalassa was convergent, whilst its southern margin facing the Mongol-Okhotsk Ocean was passive.
Central Asia
During the Cambrian, the terranes that would form Kazakhstania later in the Paleozoic were a series of island arc and accretionary complexes that lay along an intra-oceanic convergent plate margin to the south of North China.
To the south of these the Tarim microcontinent lay between Gondwana and Siberia. Its northern margin was passive for much of the Paleozoic, with thick sequences of platform carbonates and fluvial to marine sediments resting unconformably on Precambrian basement. Along its southeast margin was the Altyn Cambro–Ordovician accretionary complex, whilst to the southwest a subduction zone was closing the narrow seaway between the North West Kunlun region of Tarim and the South West Kunlun terrane.
North China

North China lay at equatorial to tropical latitudes during the early Cambrian, although its exact position is unknown. Much of the craton was covered by shallow seas, with land in the northwest and southeast.
Northern North China was a passive margin until the onset of subduction and the development of the Bainaimiao arc in the late Cambrian. To its south was a convergent margin with a southwest dipping subduction zone, beyond which lay the North Qinling terrane (now part of the Qinling Orogenic Belt).
South China and Annamia
South China and Annamia formed a single continent. Strike-slip movement between it and Gondwana accommodated its steady drift northwards from offshore the Indian sector of Gondwana to near the western Australian sector. This northward drift is evidenced by the progressive increase in limestones and increasing faunal diversity.
The northern margin South China, including the South Qinling block, was a passive margin.
Along the southeastern margin, lower Cambrian volcanics indicate the accretion of an island arc along the Song Ma suture zone. Also, early in the Cambrian, the eastern margin of South China changed from passive to active, with the development of oceanic volcanic island arcs that now form part of the Japanese terrane.
Climate
The distribution of climate-indicating sediments, including the wide latitudinal distribution of tropical carbonate platforms, archaeocyathan reefs and bauxites, and arid zone evaporites and calcrete deposits, show the Cambrian was a time of greenhouse climate conditions. During the late Cambrian the distribution of trilobite provinces also indicate only a moderate pole-to-equator temperature gradient. There is evidence of glaciation at high latitudes on Avalonia. However, it is unclear whether these sediments are early Cambrian or actually late Neoproterozoic in age.
Calculations of global average temperatures (GAT) vary depending on which techniques are used. Whilst some measurements show GAT over c. 40 °C (104 °F) models that combine multiple sources give GAT of c. 20–22 °C (68–72 °F) in the Terreneuvian increasing to c. 23–25 °C (73–77 °F) for the rest of the Cambrian. The warm climate was linked to elevated atmospheric carbon dioxide levels. Assembly of Gondwana led to the reorganisation of the tectonic plates with the development of new convergent plate margins and continental-margin arc magmatism that helped drive climatic warming. The eruptions of the Kalkarindji LIP basalts during Stage 4 and into the early Miaolingian, also released large quantities of carbon dioxide, methane and sulphur dioxide into the atmosphere leading to rapid climatic changes and elevated sea surface temperatures.
There is uncertainty around the maximum sea surface temperatures. These are calculated using δO values from marine rocks, and there is an ongoing debate about the levels δO in Cambrian seawater relative to the rest of the Phanerozoic. Estimates for tropical sea surface temperatures vary from c. 28–32 °C (82–90 °F), to c. 29–38 °C (84–100 °F). Modern average tropical sea surface temperatures are 26 °C (79 °F).
Atmospheric oxygen levels rose steadily rising from the Neoproterozoic due to the increase in photosynthesising organisms. Cambrian levels varied between c. 3% and 14% (present day levels are c. 21%). Low levels of atmospheric oxygen and the warm climate resulted in lower dissolved oxygen concentrations in marine waters and widespread anoxia in deep ocean waters.
There is a complex relationship between oxygen levels, the biogeochemistry of ocean waters, and the evolution of life. Newly evolved burrowing organisms exposed anoxic sediments to the overlying oxygenated seawater. This bioturbation decreased the burial rates of organic carbon and sulphur, which over time reduced atmospheric and oceanic oxygen levels, leading to widespread anoxic conditions. Periods of higher rates of continental weathering led to increased delivery of nutrients to the oceans, boosting productivity of phytoplankton and stimulating metazoan evolution. However, rapid increases in nutrient supply led to eutrophication, where rapid growth in phytoplankton numbers result in the depletion of oxygen in the surrounding waters.
Pulses of increased oxygen levels are linked to increased biodiversity; raised oxygen levels supported the increasing metabolic demands of organisms, and increased ecological niches by expanding habitable areas of seafloor. Conversely, incursions of oxygen-deficient water, due to changes in sea level, ocean circulation, upwellings from deeper waters and/or biological productivity, produced anoxic conditions that limited habitable areas, reduced ecological niches and resulted in extinction events both regional and global.
Overall, these dynamic, fluctuating environments, with global and regional anoxic incursions resulting in extinction events, and periods of increased oceanic oxygenation stimulating biodiversity, drove evolutionary innovation.
Geochemistry
During the Cambrian, variations in isotope ratios were more frequent and more pronounced than later in the Phanerozoic, with at least 10 carbon isotope (δC) excursions (significant variations in global isotope ratios) recognised. These excursions record changes in the biogeochemistry of the oceans and atmosphere, which are due to processes such as the global rates of continental arc magmatism, rates of weathering and nutrients levels entering the marine environment, sea level changes, and biological factors including the impact of burrowing fauna on oxygen levels.
Isotope excursions
Base of Cambrian
The basal Cambrian δC excursion (BACE), together with low δU and raised δS indicates a period of widespread shallow marine anoxia, which occurs at the same time as the extinction off the Ediacaran acritarchs. It was followed by the rapid appearance and diversification of bilaterian animals.
Cambrian Stages 2 and 3
During the early Cambrian, Sr/Sr rose in response to enhanced continental weathering. This increased the input of nutrients into the oceans and led to higher burial rates of organic matter. Over long timescales, the extra oxygen released by organic carbon burial is balanced by a decrease in the rates of pyrite (FeS2) burial (a process which also releases oxygen), leading to stable levels of oxygen in the atmosphere. However, during the early Cambrian, a series of linked δC and δS excursions indicate high burial rates of both organic carbon and pyrite in biologically productive yet anoxic ocean floor waters. The oxygen-rich waters produced by these processes spread from the deep ocean into shallow marine environments, extending the habitable regions of the seafloor. These pulses of oxygen are associated with the radiation of the small shelly fossils and the Cambrian arthropod radiation isotope excursion (CARE). The increase in oxygenated waters in the deep ocean ultimately reduced the levels of organic carbon and pyrite burial, leading to a decrease in oxygen production and the re-establishment of anoxic conditions. This cycle was repeated several times during the early Cambrian.
Cambrian Stage 4 to early Miaolingian
The beginning of the eruptions of the Kalkarindji LIP basalts during Stage 4 and the early Miaolingian released large quantities of carbon dioxide, methane and sulphur dioxide into the atmosphere. The changes these wrought are reflected by three large and rapid δC excursions. Increased temperatures led to a global sea level rise that flooded continental shelves and interiors with anoxic waters from the deeper ocean and drowned carbonate platforms of archaeocyathan reefs, resulting in the widespread accumulation of black organic-rich shales. Known as the Sinsk anoxic extinction event, this triggered the first major extinction of the Phanerozoic, the 513 - 508 Ma Botoman-Toyonian Extinction (BTE), which included the loss of the archaeocyathids and hyoliths and saw a major drop in biodiversity. The rise in sea levels is also evidenced by a global decrease in Sr/Sr. The flooding of continental areas decreased the rates of continental weathering, reducing the input of Sr to the oceans and lowering the Sr/Sr of seawater.
The base of the Miaolingian is marked by the Redlichiid–Olenellid extinction carbon isotope event (ROECE), which coincides with the main phase of Kalkarindji volcanism.
During the Miaolingian, orogenic events along the Australian-Antarctic margin of Gondwana led to an increase in weathering and an influx of nutrients into the ocean, raising the level of productivity and organic carbon burial. These can be seen in the steady increase in Sr/Sr and δC.
Early Furongian
Continued erosion of the deeper levels of the Gondwanan mountain belts led to a peak in Sr/Sr and linked positive δC and δS excursions, known as the Steptoean positive carbon isotope excursion (SPICE). This indicates similar geochemical conditions to Stages 2 and 3 of the early Cambrian existed, with the expansion of seafloor anoxia enhancing the burial rates of organic matter and pyrite. This increase in the extent of anoxic seafloor conditions led to the extinction of the marjumiid and damesellid trilobites, whilst the increase in oxygen levels that followed helped drive the radiation of plankton.
Sr/Sr fell sharply near the top of the Jiangshanian Stage, and through Stage 10 as the Gondwanan mountains were eroded down and rates of weathering decreased.
Magnesium/calcium isotope ratios in seawater
The mineralogy of inorganic marine carbonates has varied through the Phanerozoic, controlled by the Mg/Ca values of seawater. High Mg/Ca result in calcium carbonate precipitation dominated by aragonite and high-magnesium calcite, known as aragonite seas, and low ratios result in calcite seas where low-magnesium calcite is the primary calcium carbonate precipitate. The shells and skeletons of biomineralising organisms reflect the dominant form of calcite.
During the late Ediacaran to early Cambrian increasing oxygen levels led to a decrease in ocean acidity and an increase in the concentration of calcium in sea water. However, there was not a simple transition from aragonite to calcite seas, rather a protracted and variable change through the Cambrian. Aragonite and high-magnesium precipitation continued from the Ediacaran into Cambrian Stage 2. Low-magnesium calcite skeletal hard parts appear in Cambrian Age 2, but inorganic precipitation of aragonite also occurred at this time. Mixed aragonite–calcite seas continued through the middle and late Cambrian, with fully calcite seas not established until the early Ordovician.
These variations and slow decrease in Mg/Ca of seawater were due to low oxygen levels, high continental weathering rates and the geochemistry of the Cambrian seas. In conditions of low oxygen and high iron levels, iron substitutes for magnesium in authigenic clay minerals deposited on the ocean floor, slowing the removal rates of magnesium from seawater. The enrichment of ocean waters in silica, prior to the radiation of siliceous organisms, and the limited bioturbation of the anoxic ocean floor increased the rates of deposition, relative to the rest of the Phanerozoic, of these clays. This, together with the high input of magnesium into the oceans via enhanced continental weathering, delayed the reduction in Mg/Ca and facilitated continued aragonite precipitation.
The conditions that favoured the deposition of authigenic clays were also ideal for the formation of lagerstätten, with the minerals in the clays replacing the soft body parts of Cambrian organisms.
Flora
The Cambrian flora was little different from the Ediacaran. The principal taxa were the marine macroalgae Fuxianospira, Sinocylindra, and Marpolia. No calcareous macroalgae are known from the period.
No land plant (embryophyte) fossils are known from the Cambrian. However, biofilms and microbial mats were well developed on Cambrian tidal flats and beaches 500 mya, and microbes forming microbial Earth ecosystems, comparable with modern soil crust of desert regions, contributing to soil formation. Although molecular clock estimates suggest terrestrial plants may have first emerged during the Middle or Late Cambrian, the consequent large-scale removal of the greenhouse gas CO2 from the atmosphere through sequestration did not begin until the Ordovician.
Oceanic life
Life timeline | ||||||||||||||||||||||||||||||||||||||||||||||
This box: | ||||||||||||||||||||||||||||||||||||||||||||||
−4500 —–—–−4000 —–—–−3500 —–—–−3000 —–—–−2500 —–—–−2000 —–—–−1500 —–—–−1000 —–—–−500 —–—–0 — | Water Single-celled life Photosynthesis Eukaryotes Multicellular life P l a n t s Arthropods MolluscsFlowersDinosaurs MammalsBirdsPrimatesH a d e a n A r c h e a n P r o t e r o z o i cP h a n e r o z o i c |
| ||||||||||||||||||||||||||||||||||||||||||||
(million years ago)*Ice Ages |
The Cambrian explosion was a period of rapid multicellular growth. Most animal life during the Cambrian was aquatic. Trilobites were once assumed to be the dominant life form at that time, but this has proven to be incorrect. Arthropods were by far the most dominant animals in the ocean, but trilobites were only a minor part of the total arthropod diversity. What made them so apparently abundant was their heavy armor reinforced by calcium carbonate (CaCO3), which fossilized far more easily than the fragile chitinous exoskeletons of other arthropods, leaving numerous preserved remains.
The period marked a steep change in the diversity and composition of Earth's biosphere. The Ediacaran biota suffered a mass extinction at the start of the Cambrian Period, which corresponded with an increase in the abundance and complexity of burrowing behaviour. This behaviour had a profound and irreversible effect on the substrate which transformed the seabed ecosystems. Before the Cambrian, the sea floor was covered by microbial mats. By the end of the Cambrian, burrowing animals had destroyed the mats in many areas through bioturbation. As a consequence, many of those organisms that were dependent on the mats became extinct, while the other species adapted to the changed environment that now offered new ecological niches. Around the same time there was a seemingly rapid appearance of representatives of all the mineralized phyla, including the Bryozoa, which were once thought to have only appeared in the Lower Ordovician. However, many of those phyla were represented only by stem-group forms; and since mineralized phyla generally have a benthic origin, they may not be a good proxy for (more abundant) non-mineralized phyla.

While the early Cambrian showed such diversification that it has been named the Cambrian Explosion, this changed later in the period, when there occurred a sharp drop in biodiversity. About 515 Ma, the number of species going extinct exceeded the number of new species appearing. Five million years later, the number of genera had dropped from an earlier peak of about 600 to just 450. Also, the speciation rate in many groups was reduced to between a fifth and a third of previous levels. 500 Ma, oxygen levels fell dramatically in the oceans, leading to hypoxia, while the level of poisonous hydrogen sulfide simultaneously increased, causing another extinction. The later half of Cambrian was surprisingly barren and showed evidence of several rapid extinction events; the stromatolites which had been replaced by reef building sponges known as Archaeocyatha, returned once more as the archaeocyathids became extinct. This declining trend did not change until the Great Ordovician Biodiversification Event.

Some Cambrian organisms ventured onto land, producing the trace fossils Protichnites and Climactichnites. Fossil evidence suggests that euthycarcinoids, an extinct group of arthropods, produced at least some of the Protichnites. Fossils of the track-maker of Climactichnites have not been found; however, fossil trackways and resting traces suggest a large, slug-like mollusc.
In contrast to later periods, the Cambrian fauna was somewhat restricted; free-floating organisms were rare, with the majority living on or close to the sea floor; and mineralizing animals were rarer than in future periods, in part due to the unfavourable ocean chemistry.
Many modes of preservation are unique to the Cambrian, and some preserve soft body parts, resulting in an abundance of Lagerstätten. These include Sirius Passet, the Sinsk Algal Lens, the Maotianshan Shales, the Emu Bay Shale, and the Burgess Shale.
Symbol
The United States Federal Geographic Data Committee uses a "barred capital C" ⟨Ꞓ⟩ character to represent the Cambrian Period. The Unicode character is U+A792 Ꞓ LATIN CAPITAL LETTER C WITH BAR.
Gallery
-
Stromatolites of the Pika Formation (Middle Cambrian) near Helen Lake, Banff National Park, Canada
-
Trilobites, like these Elrathia kingii were very common arthropods during this time
-
Anomalocaris was an early marine predator, a member of the stem-arthropod group Radiodonta
-
Opabinia was a bizarre stem-arthropod that possessed five stalked eyes, and a fused proboscis tipped with a claw-like appendage.
-
Protichnites were the trackways of arthropods that walked Cambrian beaches
-
Hallucigenia sparsa was a member of group lobopodian, that is considered to be related to modern velvet worms.
-
Cambroraster falcatus was a hurdiid radiodont that bore a large horseshoe-shaped carapace.
-
Pikaia was a stem-chordate from the Middle Cambrian
-
Amiskwia sagittiformis was a large bodied gnathiferan from Canada and China
-
Haplophrentis was a hyolith, a group of conical shelled lophotrochozoans that were potentially related to either lophophorates or mollusks.
-
Halkieria was a bizarre invertebrate that was an early member of the mollusk group
See also
- Cambrian–Ordovician extinction event – circa 488 Ma
- Dresbachian extinction event—circa 499 Ma
- End Botomian extinction event—circa 513 Ma
- List of fossil sites (with link directory)
- Type locality (geology), the locality where a particular rock type, stratigraphic unit, fossil or mineral species is first identified
Modes of preservation in the Cambrian | ||
---|---|---|
Exceptional | ![]() | |
Conventional |
References
- ^ "International Chronostratigraphic Chart" (PDF). International Commission on Stratigraphy. December 2024. Retrieved 2 January 2025.
- Brasier, Martin; Cowie, John; Taylor, Michael (March–June 1994). "Decision on the Precambrian-Cambrian boundary stratotype" (PDF). Episodes. 17 (1–2): 3–8. doi:10.18814/epiiugs/1994/v17i1.2/002. Archived (PDF) from the original on 9 October 2022.
- Cooper, Roger; Nowlan, Godfrey; Williams, S. H. (March 2001). "Global Stratotype Section and Point for base of the Ordovician System" (PDF). Episodes. 24 (1): 19–28. doi:10.18814/epiiugs/2001/v24i1/005. Archived (PDF) from the original on 9 October 2022. Retrieved 6 December 2020.
- Haq, B. U.; Schutter, SR (2008). "A Chronology of Paleozoic Sea-Level Changes". Science. 322 (5898): 64–8. Bibcode:2008Sci...322...64H. doi:10.1126/science.1161648. PMID 18832639. S2CID 206514545.
- Howe 1911, p. 86.
- ^ Torsvik, Trond H.; Cocks, L. R. M. (2017). Earth history and palaeogeography. Cambridge, United Kingdom: Cambridge University Press. ISBN 978-1-107-10532-4. OCLC 968155663.
- ^ Myrow, Paul M.; Goodge, John W.; Brock, Glenn A.; Betts, Marissa J.; Park, Tae-Yoon S.; Hughes, Nigel C.; Gaines, Robert R. (2024). "Tectonic trigger to the first major extinction of the Phanerozoic: The early Cambrian Sinsk event". Science Advances. 10 (13): eadl3452. Bibcode:2024SciA...10L3452M. doi:10.1126/sciadv.adl3452. ISSN 2375-2548. PMC 10980278. PMID 38552008.
- ^ Pruss, Sara B.; Gill, Benjamin C. (30 May 2024). "Life on the Edge: The Cambrian Marine Realm and Oxygenation". Annual Review of Earth and Planetary Sciences. 52 (1): 109–132. doi:10.1146/annurev-earth-031621-070316. hdl:10919/117422. ISSN 0084-6597.
- Butterfield, N. J. (2007). "Macroevolution and macroecology through deep time". Palaeontology. 50 (1): 41–55. Bibcode:2007Palgy..50...41B. doi:10.1111/j.1475-4983.2006.00613.x.
- Orr, P. J.; Benton, M. J.; Briggs, D. E. G. (2003). "Post-Cambrian closure of the deep-water slope-basin taphonomic window". Geology. 31 (9): 769–772. Bibcode:2003Geo....31..769O. doi:10.1130/G19193.1.
- Collette, Gass & Hagadorn 2012.
- Edgecombe, Gregory D.; Strullu-Derrien, Christine; Góral, Tomasz; Hetherington, Alexander J.; Thompson, Christine; Koch, Marcus (2020). "Aquatic stem group myriapods close a gap between molecular divergence dates and terrestrial fossil record". Proceedings of the National Academy of Sciences. 117 (16): 8966–8972. Bibcode:2020PNAS..117.8966E. doi:10.1073/pnas.1920733117. PMC 7183169. PMID 32253305. S2CID 215408474.
- Lozano-Fernandez, Jesus; Tanner, Alastair R.; Puttick, Mark N.; Vinther, Jakob; Edgecombe, Gregory D.; Pisani, Davide (2020). "A Cambrian–Ordovician Terrestrialization of Arachnids". Frontiers in Genetics. 11: 182. doi:10.3389/fgene.2020.00182. PMC 7078165. PMID 32218802.
- Lozano-Fernandez, Jesus; Carton, Robert; Tanner, Alastair R.; Puttick, Mark N.; Blaxter, Mark; Vinther, Jakob; Olesen, Jørgen; Giribet, Gonzalo; Edgecombe, Gregory D.; Pisani, Davide (2016). "A molecular palaeobiological exploration of arthropod terrestrialization". Philosophical Transactions of the Royal Society B: Biological Sciences. 371 (1699). doi:10.1098/rstb.2015.0133. PMC 4920334. PMID 27325830.
- De Vries, Jan; De Vries, Sophie; Fürst-Jansen, Janine M R. (2020). "Evo-physio: On stress responses and the earliest land plants". Journal of Experimental Botany. 71 (11): 3254–3269. doi:10.1093/jxb/eraa007. PMC 7289718. PMID 31922568.
- Morris, Jennifer L.; Puttick, Mark N.; Clark, James W.; Edwards, Dianne; Kenrick, Paul; Pressel, Silvia; Wellman, Charles H.; Yang, Ziheng; Schneider, Harald; Donoghue, Philip C. J. (2018). "The timescale of early land plant evolution". Proceedings of the National Academy of Sciences. 115 (10): E2274 – E2283. Bibcode:2018PNAS..115E2274M. doi:10.1073/pnas.1719588115. PMC 5877938. PMID 29463716.
- ^ Peng, S.C.; Babcock, L.E.; Ahlberg, P. (2020), "The Cambrian Period", Geologic Time Scale 2020, Elsevier, pp. 565–629, doi:10.1016/b978-0-12-824360-2.00019-x, ISBN 978-0-12-824360-2, S2CID 242177216, retrieved 8 June 2023
- ^ Davidson, Nick (2021). The Greywacke (1st ed.). London: Profile Books Ltd. ISBN 9781788163781.
- Sedgwick, A. (1852). "On the classification and nomenclature of the Lower Paleozoic rocks of England and Wales". Q. J. Geol. Soc. Lond. 8 (1–2): 136–138. doi:10.1144/GSL.JGS.1852.008.01-02.20. S2CID 130896939.
- ^ Geyer, Gerd; Landing, Ed (2016). "The Precambrian–Phanerozoic and Ediacaran–Cambrian boundaries: A historical approach to a dilemma". Geological Society, London, Special Publications. 448 (1): 311–349. Bibcode:2017GSLSP.448..311G. doi:10.1144/SP448.10. S2CID 133538050.
- Landing, Ed; Geyer, Gerd; Brasier, Martin D.; Bowring, Samuel A. (2013). "Cambrian Evolutionary Radiation: Context, correlation, and chronostratigraphy—Overcoming deficiencies of the first appearance datum (FAD) concept". Earth-Science Reviews. 123: 133–172. Bibcode:2013ESRv..123..133L. doi:10.1016/j.earscirev.2013.03.008.
- A.Yu. Rozanov; V.V. Khomentovsky; Yu.Ya. Shabanov; G.A. Karlova; A.I. Varlamov; V.A. Luchinina; T.V. Pegel'; Yu.E. Demidenko; P.Yu. Parkhaev; I.V. Korovnikov; N.A. Skorlotova (2008). "To the problem of stage subdivision of the Lower Cambrian". Stratigraphy and Geological Correlation. 16 (1): 1–19. Bibcode:2008SGC....16....1R. doi:10.1007/s11506-008-1001-3. S2CID 128128572.
- B. S. Sokolov; M. A. Fedonkin (1984). "The Vendian as the Terminal System of the Precambrian". Episodes. 7 (1): 12–20. doi:10.18814/epiiugs/1984/v7i1/004.
- V. V. Khomentovskii; G. A. Karlova (2005). "The Tommotian Stage Base as the Cambrian Lower Boundary in Siberia". Stratigraphy and Geological Correlation. 13 (1): 21–34. Archived from the original on 14 July 2011. Retrieved 15 March 2009.
- Nielsen, Arne Thorshøj (3 October 2019). "The Miaolingian, a new name for the 'Middle' Cambrian (Cambrian Series 3): identification of lower and upper boundaries in Baltoscandia". GFF. 141 (2): 162–173. doi:10.1080/11035897.2019.1621374. Retrieved 2 January 2025.
- ^ Zhao, Yuanlong; Yuan, Jinliang; Babcock, Loren E.; Guo, Qingjun; Peng, Jin; Yin, Leiming; Yang, Xinglian; Peng, Shanchi; Wang, Chunjiang; Gaines, Robert R.; Esteve, Jorge; Tai, Tongsu; Yang, Ruidong; Wang, Yue; Sun, Haijing (1 June 2019). "Global Standard Stratotype-Section and Point (GSSP) for the conterminous base of the Miaolingian Series and Wuliuan Stage (Cambrian) at Balang, Jianhe, Guizhou, China". Episodes Journal of International Geoscience. 42 (2): 165–184. doi:10.18814/epiiugs/2019/019013.
- Cooper, R.A.; Sadler, P.M.; Hammer, O.; Gradstein, F.M. (2012), "The Ordovician Period", The Geologic Time Scale, Elsevier, pp. 489–523, doi:10.1016/b978-0-444-59425-9.00020-2, ISBN 978-0-444-59425-9, retrieved 12 May 2024
- Suuroja, K; Suuroja, S (2010). "The Neugrund meteorite crater on the seafloor of the Gulf of Finland". Baltica. 23 (1): 47–58.
- Kalleson, Elin (2009). "The Gardnos structure : the impactites, sedimentary deposits and post-impact history" (PDF).
- "Earth impact database". www.passc.net. Retrieved 20 September 2024.
- ^ Keppie, Duncan Fraser; Keppie, John Duncan; Landing, Ed (23 April 2024). "A tectonic solution for the Early Cambrian palaeogeographical enigma". Geological Society, London, Special Publications. 542 (1): 167–177. doi:10.1144/SP542-2022-355. ISSN 0305-8719.
- Domeier, Mathew (1 August 2016). "A plate tectonic scenario for the Iapetus and Rheic oceans". Gondwana Research. 36: 275–295. doi:10.1016/j.gr.2015.08.003. ISSN 1342-937X.
- Dalziel, Ian W. D.; Dewey, John F. (2019). "The classic Wilson cycle revisited". Geological Society, London, Special Publications. 470 (1): 19–38. Bibcode:2019GSLSP.470...19D. doi:10.1144/SP470.1. ISSN 0305-8719.
- ^ Wong Hearing, Thomas W.; Pohl, Alexandre; Williams, Mark; Donnadieu, Yannick; Harvey, Thomas H. P.; Scotese, Christopher R.; Sepulchre, Pierre; Franc, Alain; Vandenbroucke, Thijs R. A. (23 June 2021). "Quantitative comparison of geological data and model simulations constrains early Cambrian geography and climate". Nature Communications. 12 (1): 3868. Bibcode:2021NatCo..12.3868W. doi:10.1038/s41467-021-24141-5. hdl:1854/LU-8719399. ISSN 2041-1723. PMC 8222365. PMID 34162853.
- ^ Domeier, Mathew (2016). "A plate tectonic scenario for the Iapetus and Rheic oceans". Gondwana Research. 36: 275–295. Bibcode:2016GondR..36..275D. doi:10.1016/j.gr.2015.08.003.
- Evans, David A. D. (2021). "Pannotia under prosecution". Geological Society, London, Special Publications. 503 (1): 63–81. Bibcode:2021GSLSP.503...63E. doi:10.1144/SP503-2020-182. ISSN 0305-8719.
- Grantham, Geoffrey H.; Satish-Kumar, M.; Horie, Kenji; Ueckermann, Henriette (2023). "The Kuunga Accretionary Complex of Sverdrupfjella and Gjelsvikfjella, western Dronning Maud Land, Antarctica". Journal of Mineralogical and Petrological Sciences. 118 (ANTARCTICA): 230125. doi:10.2465/jmps.230125.
- ^ Murphy, J. Brendan; Nance, R. Damian; Cawood, Peter A.; Collins, William J.; Dan, Wei; Doucet, Luc S.; Heron, Philip J.; Li, Zheng-Xiang; Mitchell, Ross N.; Pisarevsky, Sergei; Pufahl, Peir K.; Quesada, Cecilio; Spencer, Christopher J.; Strachan, Rob A.; Wu, Lei (2021). "Pannotia: in defence of its existence and geodynamic significance". Geological Society, London, Special Publications. 503 (1): 13–39. Bibcode:2021GSLSP.503...13M. doi:10.1144/SP503-2020-96. hdl:20.500.11937/90589. ISSN 0305-8719.
- Domeier, Mathew (1 May 2018). "Early Paleozoic tectonics of Asia: Towards a full-plate model". Geoscience Frontiers. Greenstone belts and their mineral endowment. 9 (3): 789–862. doi:10.1016/j.gsf.2017.11.012. hdl:10852/71215. ISSN 1674-9871.
- ^ Domeier, Mathew (2018). "Early Paleozoic tectonics of Asia: Towards a full-plate model". Geoscience Frontiers. 9 (3): 789–862. Bibcode:2018GeoFr...9..789D. doi:10.1016/j.gsf.2017.11.012. hdl:10852/71215. ISSN 1674-9871.
- ^ Hearing, Thomas W.; Harvey, Thomas H. P.; Williams, Mark; Leng, Melanie J.; Lamb, Angela L.; Wilby, Philip R.; Gabbott, Sarah E.; Pohl, Alexandre; Donnadieu, Yannick (9 May 2018). "An early Cambrian greenhouse climate". Science Advances. 4 (5): eaar5690. Bibcode:2018SciA....4.5690H. doi:10.1126/sciadv.aar5690. PMC 5942912. PMID 29750198.
- ^ Scotese, Christopher R.; Song, Haijun; Mills, Benjamin J.W.; van der Meer, Douwe G. (April 2021). "Phanerozoic paleotemperatures: The earth's changing climate during the last 540 million years". Earth-Science Reviews. 215: 103503. Bibcode:2021ESRv..21503503S. doi:10.1016/j.earscirev.2021.103503. ISSN 0012-8252. S2CID 233579194. Archived from the original on 8 January 2021. Alt URL
- ^ Wotte, Thomas; Skovsted, Christian B.; Whitehouse, Martin J.; Kouchinsky, Artem (19 April 2019). "Isotopic evidence for temperate oceans during the Cambrian Explosion". Scientific Reports. 9 (1): 6330. Bibcode:2019NatSR...9.6330W. doi:10.1038/s41598-019-42719-4. ISSN 2045-2322. PMC 6474879. PMID 31004083.
- Bergmann, Kristin D.; Finnegan, Seth; Creel, Roger; Eiler, John M.; Hughes, Nigel C.; Popov, Leonid E.; Fischer, Woodward W. (2018). "A paired apatite and calcite clumped isotope thermometry approach to estimating Cambro-Ordovician seawater temperatures and isotopic composition". Geochimica et Cosmochimica Acta. 224: 18–41. Bibcode:2018GeCoA.224...18B. doi:10.1016/j.gca.2017.11.015. ISSN 0016-7037.
- ^ Mills, Benjamin J.W.; Krause, Alexander J.; Jarvis, Ian; Cramer, Bradley D. (31 May 2023). "Evolution of Atmospheric O 2 Through the Phanerozoic, Revisited". Annual Review of Earth and Planetary Sciences. 51 (1): 253–276. doi:10.1146/annurev-earth-032320-095425. ISSN 0084-6597.
- ^ van de Velde, Sebastiaan; Mills, Benjamin J. W.; Meysman, Filip J. R.; Lenton, Timothy M.; Poulton, Simon W. (2 July 2018). "Early Palaeozoic ocean anoxia and global warming driven by the evolution of shallow burrowing". Nature Communications. 9 (1): 2554. Bibcode:2018NatCo...9.2554V. doi:10.1038/s41467-018-04973-4. ISSN 2041-1723. PMC 6028391. PMID 29967319.
- ^ Wood, Rachel; Liu, Alexander G.; Bowyer, Frederick; Wilby, Philip R.; Dunn, Frances S.; Kenchington, Charlotte G.; Cuthill, Jennifer F. Hoyal; Mitchell, Emily G.; Penny, Amelia (2019). "Integrated records of environmental change and evolution challenge the Cambrian Explosion". Nature Ecology & Evolution. 3 (4): 528–538. Bibcode:2019NatEE...3..528W. doi:10.1038/s41559-019-0821-6. ISSN 2397-334X. PMID 30858589.
- ^ Zhang, Yinggang; Yang, Tao; Hohl, Simon V.; Zhu, Bi; He, Tianchen; Pan, Wenqing; Chen, Yongquan; Yao, Xizhu; Jiang, Shaoyong (2020). "Seawater carbon and strontium isotope variations through the late Ediacaran to late Cambrian in the Tarim Basin". Precambrian Research. 345: 105769. Bibcode:2020PreR..34505769Z. doi:10.1016/j.precamres.2020.105769. ISSN 0301-9268.
- ^ He, Tianchen; Zhu, Maoyan; Mills, Benjamin J. W.; Wynn, Peter M.; Zhuravlev, Andrey Yu; Tostevin, Rosalie; Pogge von Strandmann, Philip A. E.; Yang, Aihua; Poulton, Simon W.; Shields, Graham A. (2019). "Possible links between extreme oxygen perturbations and the Cambrian radiation of animals". Nature Geoscience. 12 (6): 468–474. Bibcode:2019NatGe..12..468H. doi:10.1038/s41561-019-0357-z. ISSN 1752-0908. PMC 6548555. PMID 31178922.
- ^ Wei, Guang-Yi; Hood, Ashleigh v. S.; Planavsky, Noah J.; Li, Da; Ling, Hong-Fei; Tarhan, Lidya G. (2022). "Calcium Isotopic Constraints on the Transition From Aragonite Seas to Calcite Seas in the Cambrian". Global Biogeochemical Cycles. 36 (5). Bibcode:2022GBioC..3607235W. doi:10.1029/2021GB007235. ISSN 0886-6236.
- ^ Xiong, Yi; Wood, Rachel; Pichevin, Laetitia (2023). "The record of sea water chemistry evolution during the Ediacaran–Cambrian from early marine cements". The Depositional Record. 9 (3): 508–525. Bibcode:2023DepRe...9..508X. doi:10.1002/dep2.211. hdl:20.500.11820/3e22be46-182d-421a-a791-8a20a96c9814. ISSN 2055-4877.
- LoDuca, S. T.; Bykova, N.; Wu, M.; Xiao, S.; Zhao, Y. (July 2017). "Seaweed morphology and ecology during the great animal diversification events of the early Paleozoic: A tale of two floras". Geobiology. 15 (4): 588–616. Bibcode:2017Gbio...15..588L. doi:10.1111/gbi.12244. PMID 28603844.
- Schieber et al. 2007, pp. 53–71.
- Retallack, G.J. (2008). "Cambrian palaeosols and landscapes of South Australia". Alcheringa. 55 (8): 1083–1106. Bibcode:2008AuJES..55.1083R. doi:10.1080/08120090802266568. S2CID 128961644.
- "Greening of the Earth pushed way back in time". Phys.org. University of Oregon. 22 July 2013.
- Donoghue, Philip C.J.; Harrison, C. Jill; Paps, Jordi; Schneider, Harald (October 2021). "The evolutionary emergence of land plants". Current Biology. 31 (19): R1281 – R1298. Bibcode:2021CBio...31R1281D. doi:10.1016/j.cub.2021.07.038. hdl:1983/662d176e-fcf4-40bf-aa8c-5694a86bd41d. PMID 34637740. S2CID 238588736.
- Paselk, Richard (28 October 2012). "Cambrian". Natural History Museum. Humboldt State University.
- Ward, Peter (2006). 3 Evolving Respiratory Systems as a Cause of the Cambrian Explosion – Out of Thin Air: Dinosaurs, Birds, and Earth's Ancient Atmosphere – The National Academies Press. doi:10.17226/11630. ISBN 978-0-309-10061-8.
- Perkins, Sid (23 October 2009). "As the worms churn". ScienceNews. Archived from the original on 25 October 2009.
- Zhang, Zhiliang; Zhang, Zhifei; Ma, J.; Taylor, P. D.; Strotz, L. C.; Jacquet, S. M.; Skovsted, C. B.; Chen, F.; Han, J.; Brock, G. A. (2021). "Fossil evidence unveils an early Cambrian origin for Bryozoa". Nature. 599 (7884): 251–255. Bibcode:2021Natur.599..251Z. doi:10.1038/s41586-021-04033-w. PMC 8580826. PMID 34707285. S2CID 240073948.
- Taylor, P.D.; Berning, B.; Wilson, M.A. (2013). "Reinterpretation of the Cambrian 'bryozoan' Pywackia as an octocoral". Journal of Paleontology. 87 (6): 984–990. Bibcode:2013JPal...87..984T. doi:10.1666/13-029. S2CID 129113026.
- Budd, G. E.; Jensen, S. (2000). "A critical reappraisal of the fossil record of the bilaterian phyla". Biological Reviews of the Cambridge Philosophical Society. 75 (2): 253–95. doi:10.1111/j.1469-185X.1999.tb00046.x. PMID 10881389. S2CID 39772232.
- Nanglu, Karma; Caron, Jean-Bernard; Conway Morris, Simon; Cameron, Christopher B. (2016). "Cambrian suspension-feeding tubicolous hemichordates". BMC Biology. 14: 56. doi:10.1186/s12915-016-0271-4. PMC 4936055. PMID 27383414.
- "The Ordovician: Life's second big bang". Archived from the original on 9 October 2018. Retrieved 10 February 2013.
- Marshall, Michael. "Oxygen crash led to Cambrian mass extinction".
- Collette & Hagadorn 2010; Collette, Gass & Hagadorn 2012.
- Yochelson & Fedonkin 1993; Getty & Hagadorn 2008.
- ^ Munnecke, A.; Calner, M.; Harper, D. A. T.; Servais, T. (2010). "Ordovician and Silurian sea-water chemistry, sea level, and climate: A synopsis". Palaeogeography, Palaeoclimatology, Palaeoecology. 296 (3–4): 389–413. Bibcode:2010PPP...296..389M. doi:10.1016/j.palaeo.2010.08.001.
- Strang, Katie M.; Armstrong, Howard A.; Harper, David A. T.; Trabucho-Alexandre, João P. (2016). "The Sirius Passet Lagerstätte: Silica death masking opens the window on the earliest matground community of the Cambrian explosion". Lethaia. 49 (4): 631–643. doi:10.1111/let.12174.
- Nielsen, Morten Lunde; Lee, Mirinae; Ng, Hong Chin; Rushton, Jeremy C.; Hendry, Katharine R.; Kihm, Ji-Hoon; Nielsen, Arne T.; Park, Tae-Yoon S.; Vinther, Jakob; Wilby, Philip R. (1 January 2022). "Metamorphism obscures primary taphonomic pathways in the early Cambrian Sirius Passet Lagerstätte, North Greenland". Geology. 50 (1): 4–9. Bibcode:2022Geo....50....4N. doi:10.1130/G48906.1. ISSN 0091-7613.
- Ivantsov, Andrey Yu.; Zhuravlev, Andrey Yu.; Leguta, Anton V.; Krassilov, Valentin A.; Melnikova, Lyudmila M.; Ushatinskaya, Galina T. (2 May 2005). "Palaeoecology of the Early Cambrian Sinsk biota from the Siberian Platform". Palaeogeography, Palaeoclimatology, Palaeoecology. 220 (1–2): 69–88. Bibcode:2005PPP...220...69I. doi:10.1016/j.palaeo.2004.01.022. Retrieved 12 November 2022.
- MacKenzie, Lindsay A.; Hofmann, Michael H.; Junyuan, Chen; Hinman, Nancy W. (15 February 2015). "Stratigraphic controls of soft-bodied fossil occurrences in the Cambrian Chengjiang Biota Lagerstätte, Maotianshan Shale, Yunnan Province, China". Palaeogeography, Palaeoclimatology, Palaeoecology. 420: 96–115. Bibcode:2015PPP...420...96M. doi:10.1016/j.palaeo.2014.11.006. Retrieved 12 November 2022.
- Paterson, John R.; García-Bellido, Diego C.; Jago, James B.; Gehling, James G.; Lee, Michael S. Y.; Edgecombe, Gregory D. (10 November 2015). "The Emu Bay Shale Konservat-Lagerstätte: a view of Cambrian life from East Gondwana". Journal of the Geological Society. 173 (1): 1–11. doi:10.1144/jgs2015-083. S2CID 130614466. Retrieved 12 November 2022.
- Butterfield, N.J. (1990). "Organic Preservation of Non-Mineralizing Organisms and the Taphonomy of the Burgess Shale". Paleobiology. 16 (3): 272–286. Bibcode:1990Pbio...16..272B. doi:10.1017/S0094837300009994. JSTOR 2400788. S2CID 133486523.
- Page, Alex; Gabbott, Sarah; Wilby, Phillip R.; Zalasiewicz, Jan A. (2008). "Ubiquitous Burgess Shale–style "clay templates" in low-grade metamorphic mudrocks". Geology. 36 (11): 855–858. Bibcode:2008Geo....36..855P. doi:10.1130/G24991A.1.
- Orr, Patrick J.; Briggs, Derek E. G.; Kearns, Stuart L. (1998). "Cambrian Burgess Shale Animals Replicated in Clay Minerals". Science. 281 (5380): 1173–5. Bibcode:1998Sci...281.1173O. doi:10.1126/science.281.5380.1173. PMID 9712577.
- Federal Geographic Data Committee, ed. (August 2006). FGDC Digital Cartographic Standard for Geologic Map Symbolization FGDC-STD-013-2006 (PDF). U.S. Geological Survey for the Federal Geographic Data Committee. p. A–32–1. Archived (PDF) from the original on 9 October 2022. Retrieved 23 August 2010.
- Priest, Lorna A.; Iancu, Laurentiu; Everson, Michael (October 2010). "Proposal to Encode C WITH BAR" (PDF). Archived (PDF) from the original on 9 October 2022. Retrieved 6 April 2011.
- Unicode Character 'LATIN CAPITAL LETTER C WITH BAR' (U+A792). fileformat.info. Retrieved 15 June 2015
Further reading
- Amthor, J. E.; Grotzinger, John P.; Schröder, Stefan; Bowring, Samuel A.; Ramezani, Jahandar; Martin, Mark W.; Matter, Albert (2003). "Extinction of Cloudina and Namacalathus at the Precambrian-Cambrian boundary in Oman". Geology. 31 (5): 431–434. Bibcode:2003Geo....31..431A. doi:10.1130/0091-7613(2003)031<0431:EOCANA>2.0.CO;2.
- Collette, J. H.; Gass, K. C.; Hagadorn, J. W. (2012). "Protichnites eremita unshelled? Experimental model-based neoichnology and new evidence for a euthycarcinoid affinity for this ichnospecies". Journal of Paleontology. 86 (3): 442–454. Bibcode:2012JPal...86..442C. doi:10.1666/11-056.1. S2CID 129234373.
- Collette, J. H.; Hagadorn, J. W. (2010). "Three-dimensionally preserved arthropods from Cambrian Lagerstatten of Quebec and Wisconsin". Journal of Paleontology. 84 (4): 646–667. doi:10.1666/09-075.1. S2CID 130064618.
- Getty, P. R.; Hagadorn, J. W. (2008). "Reinterpretation of Climactichnites Logan 1860 to include subsurface burrows, and erection of Musculopodus for resting traces of the trailmaker". Journal of Paleontology. 82 (6): 1161–1172. Bibcode:2008JPal...82.1161G. doi:10.1666/08-004.1. S2CID 129732925.
- Gould, S. J. (1989). Wonderful Life: the Burgess Shale and the Nature of Life. New York: Norton. ISBN 9780393027051.
- Howe, John Allen (1911). "Cambrian System" . In Chisholm, Hugh (ed.). Encyclopædia Britannica. Vol. 05 (11th ed.). Cambridge University Press. pp. 86–89.
- Ogg, J. (June 2004). "Overview of Global Boundary Stratotype Sections and Points (GSSPs)". Archived from the original on 23 April 2006. Retrieved 30 April 2006.
- Owen, R. (1852). "Description of the impressions and footprints of the Protichnites from the Potsdam sandstone of Canada". Geological Society of London Quarterly Journal. 8 (1–2): 214–225. doi:10.1144/GSL.JGS.1852.008.01-02.26. S2CID 130712914.
- Peng, S.; Babcock, L.E.; Cooper, R.A. (2012). "The Cambrian Period" (PDF). The Geologic Time Scale. Archived from the original (PDF) on 12 February 2015. Retrieved 14 January 2015.
- Schieber, J.; Bose, P. K.; Eriksson, P. G.; Banerjee, S.; Sarkar, S.; Altermann, W.; Catuneau, O. (2007). Atlas of Microbial Mat Features Preserved within the Clastic Rock Record. Elsevier. pp. 53–71. ISBN 9780444528599.
- Yochelson, E. L.; Fedonkin, M. A. (1993). "Paleobiology of Climactichnites, and Enigmatic Late Cambrian Fossil". Smithsonian Contributions to Paleobiology. 74 (74): 1–74. doi:10.5479/si.00810266.74.1.
External links
- Cambrian period on In Our Time at the BBC
- Biostratigraphy – includes information on Cambrian trilobite biostratigraphy
- Sam Gon's trilobite pages (contains numerous Cambrian trilobites)
- Examples of Cambrian Fossils
- Paleomap Project
- Report on the web on Amthor and others from Geology vol. 31
- Weird Life on the Mats
- Chronostratigraphy scale v.2018/08 | Cambrian
Cambrian Period | |||||||||
---|---|---|---|---|---|---|---|---|---|
|