| |||
| |||
Names | |||
---|---|---|---|
Preferred IUPAC name Propene | |||
Identifiers | |||
CAS Number | |||
3D model (JSmol) | |||
Beilstein Reference | 1696878 | ||
ChEBI | |||
ChEMBL | |||
ChemSpider | |||
ECHA InfoCard | 100.003.693 ![]() | ||
EC Number |
| ||
Gmelin Reference | 852 | ||
KEGG | |||
PubChem CID | |||
RTECS number |
| ||
UNII | |||
UN number | 1077 In Liquefied petroleum gas: 1075 | ||
CompTox Dashboard (EPA) | |||
InChI
| |||
SMILES
| |||
Properties | |||
Chemical formula | C3H6 | ||
Molar mass | 42.081 g·mol | ||
Appearance | Colorless gas | ||
Density | 1.81 kg/m, gas (1.013 bar, 15 °C) 1.745 kg/m, gas (1.013 bar, 25 °C) 613.9 kg/m, liquid | ||
Melting point | −185.2 °C (−301.4 °F; 88.0 K) | ||
Boiling point | −47.6 °C (−53.7 °F; 225.6 K) | ||
Solubility in water | 0.61 g/m | ||
Magnetic susceptibility (χ) | -31.5·10 cm/mol | ||
Viscosity | 8.34 µPa·s at 16.7 °C | ||
Structure | |||
Dipole moment | 0.366 D (gas) | ||
Hazards | |||
GHS labelling: | |||
Pictograms | ![]() | ||
Signal word | Danger | ||
Hazard statements | H220 | ||
Precautionary statements | P210, P377, P381, P403 | ||
NFPA 704 (fire diamond) |
![]() | ||
Flash point | −108 °C (−162 °F; 165 K) | ||
Safety data sheet (SDS) | External MSDS | ||
Related compounds | |||
Related alkenes; related groups |
Ethylene, Isomers of Butylene; Allyl, Propenyl | ||
Related compounds | Propane, Propyne Propadiene, 1-Propanol 2-Propanol | ||
Except where otherwise noted, data are given for materials in their standard state (at 25 °C , 100 kPa).
![]() |
Propylene, also known as propene, is an unsaturated organic compound with the chemical formula CH3CH=CH2. It has one double bond, and is the second simplest member of the alkene class of hydrocarbons. It is a colorless gas with a faint petroleum-like odor.
Propylene is a product of combustion from forest fires, cigarette smoke, and motor vehicle and aircraft exhaust. It was discovered in 1850 by A. W. von Hoffman's student Captain (later Major General) John Williams Reynolds as the only gaseous product of thermal decomposition of amyl alcohol to react with chlorine and bromine.
Production
Steam cracking
Main article: Steam crackingThe dominant technology for producing propylene is steam cracking, using propane as the feedstock. Cracking propane yields a mixture of ethylene, propylene, methane, hydrogen gas, and other related compounds. The yield of propylene is about 15%. The other principal feedstock is naphtha, especially in the Middle East and Asia. Propylene can be separated by fractional distillation from the hydrocarbon mixtures obtained from cracking and other refining processes; refinery-grade propene is about 50 to 70%. In the United States, shale gas is a major source of propane.
Olefin conversion technology
In the Phillips triolefin or olefin conversion technology, propylene is interconverted with ethylene and 2-butenes. Rhenium and molybdenum catalysts are used:
The technology is founded on an olefin metathesis reaction discovered at Phillips Petroleum Company. Propylene yields of about 90 wt% are achieved.
Main article: Syngas to gasoline plusRelated is the Methanol-to-Olefins/Methanol-to-Propene process. It converts synthesis gas (syngas) to methanol, and then converts the methanol to ethylene and/or propene. The process produces water as a by-product. Synthesis gas is produced from the reformation of natural gas or by the steam-induced reformation of petroleum products such as naphtha, or by gasification of coal or natural gas.
Fluid catalytic cracking
High severity fluid catalytic cracking (FCC) uses traditional FCC technology under severe conditions (higher catalyst-to-oil ratios, higher steam injection rates, higher temperatures, etc.) in order to maximize the amount of propene and other light products. A high severity FCC unit is usually fed with gas oils (paraffins) and residues, and produces about 20–25% (by mass) of propene on feedstock together with greater volumes of motor gasoline and distillate byproducts. These high temperature processes are expensive and have a high carbon footprint. For these reasons, alternative routes to propylene continue to attract attention.
Other commercialized methods
On-purpose propylene production technologies were developed throughout the twentieth century. Of these, propane dehydrogenation technologies such as the CATOFIN and OLEFLEX processes have become common, although they still make up a minority of the market, with most of the olefin being sourced from the above mentioned cracking technologies. Platinum, chromia, and vanadium catalysts are common in propane dehydrogenation processes.
Market
Propene production has remained static at around 35 million tonnes (Europe and North America only) from 2000 to 2008, but it has been increasing in East Asia, most notably Singapore and China. Total world production of propene is currently about half that of ethylene.
Research
The use of engineered enzymes has been explored but has not been commercialized.
There is ongoing research into the use of oxygen carrier catalysts for the oxidative dehydrogenation of propane. This poses several advantages, as this reaction mechanism can occur at lower temperatures than conventional dehydrogenation, and may not be equilibrium-limited because oxygen is used to combust the hydrogen by-product.
Uses
Propene is the second most important starting product in the petrochemical industry after ethylene. It is the raw material for a wide variety of products. Polypropylene manufacturers consume nearly two thirds of global production. Polypropylene end uses include films, fibers, containers, packaging, and caps and closures. Propene is also used for the production of important chemicals such as propylene oxide, acrylonitrile, cumene, butyraldehyde, and acrylic acid. In the year 2013 about 85 million tonnes of propene were processed worldwide.
Propene and benzene are converted to acetone and phenol via the cumene process.
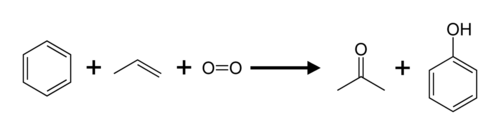
Propene is also used to produce isopropyl alcohol (propan-2-ol), acrylonitrile, propylene oxide, and epichlorohydrin. The industrial production of acrylic acid involves the catalytic partial oxidation of propene. Propylene is an intermediate in the oxidation to acrylic acid.
In industry and workshops, propene is used as an alternative fuel to acetylene in Oxy-fuel welding and cutting, brazing and heating of metal for the purpose of bending. It has become a standard in BernzOmatic products and others in MAPP substitutes, now that true MAPP gas is no longer available.
Reactions
Propene resembles other alkenes in that it undergoes addition reactions relatively easily at room temperature. The relative weakness of its double bond explains its tendency to react with substances that can achieve this transformation. Alkene reactions include: 1) polymerization, 2) oxidation, 3) halogenation and hydrohalogenation, 4) alkylation, 5) hydration, 6) oligomerization, and 7) hydroformylation.
Complexes of transition metals
Foundational to hydroformylation, alkene metathesis, and polymerization are metal-propylene complexes, which are intermediates in these processes. Propylene is prochiral, meaning that binding of a reagent (such as a metal electrophile) to the C=C group yields one of two enantiomers.
Polymerization
See also: PolypropyleneThe majority of propene is used to form polypropylene, a very important commodity thermoplastic, through chain-growth polymerization. In the presence of a suitable catalyst (typically a Ziegler–Natta catalyst), propene will polymerize. There are multiple ways to achieve this, such as using high pressures to suspending the catalyst in a solution of liquid propene, or running gaseous propene through a fluidized bed reactor.
Oligomerizationn
In the presence of catalysts, propylene will form various short oligomers. It can dimerizes to give 2,3-dimethyl-1-butene and/or 2,3-dimethyl-2-butene. or trimerise to form tripropylene.
Environmental safety
Propene is a product of combustion from forest fires, cigarette smoke, and motor vehicle and aircraft exhaust. It is an impurity in some heating gases. Observed concentrations have been in the range of 0.1–4.8 parts per billion (ppb) in rural air, 4–10.5 ppb in urban air, and 7–260 ppb in industrial air samples.
In the United States and some European countries a threshold limit value of 500 parts per million (ppm) was established for occupational (8-hour time-weighted average) exposure. It is considered a volatile organic compound (VOC) and emissions are regulated by many governments, but it is not listed by the U.S. Environmental Protection Agency (EPA) as a hazardous air pollutant under the Clean Air Act. With a relatively short half-life, it is not expected to bioaccumulate.
Propene has low acute toxicity from inhalation and is not considered to be carcinogenic. Chronic toxicity studies in mice did not yield significant evidence suggesting adverse effects. Humans briefly exposed to 4,000 ppm did not experience any noticeable effects. Propene is dangerous from its potential to displace oxygen as an asphyxiant gas, and from its high flammability/explosion risk.
Bio-propylene is the bio-based propylene. It has been examined, motivated by diverse interests such a carbon footprint. Production from glucose has been considered. More advanced ways of addressing such issues focus on electrification alternatives to steam cracking.
Storage and handling
Propene is flammable. Propene is usually stored as liquid under pressure, although it is also possible to store it safely as gas at ambient temperature in approved containers.
Occurrence in nature
Propene is detected in the interstellar medium through microwave spectroscopy. On September 30, 2013, NASA also announced that the Cassini orbiter spacecraft, part of the Cassini-Huygens mission, had discovered small amounts of naturally occurring propene in the atmosphere of Titan using spectroscopy.
See also
References
- "General Principles, Rules, and Conventions". Nomenclature of Organic Chemistry: IUPAC Recommendations and Preferred Names 2013 (Blue Book). Cambridge: The Royal Society of Chemistry. 2014. p. 31. doi:10.1039/9781849733069-00001. ISBN 978-0-85404-182-4.
- Moss, G.P. (web version). "P-14.3 Locants". Nomenclature of Organic Chemistry. IUPAC Recommendations and Preferred Names 2013. London: Queen Mary University. Section P-14.3.4.2 (d). Retrieved 23 August 2024.
- "Propylene". pubchem.ncbi.nlm.nih.gov. Retrieved 14 December 2021.
- "Propylene".
- ^ Morgott, David (2018-01-04). "The Human Exposure Potential from Propylene Releases to the Environment". International Journal of Environmental Research and Public Health. 15 (1): 66. doi:10.3390/ijerph15010066. ISSN 1660-4601. PMC 5800165. PMID 29300328.
- "Maj Gen John Williams Reynolds, FCS". geni_family_tree. 1816-12-25. Retrieved 2023-12-30.
- Rasmussen, Seth C. (2018), Rasmussen, Seth C. (ed.), "Introduction", Acetylene and Its Polymers: 150+ Years of History, SpringerBriefs in Molecular Science, Cham: Springer International Publishing, pp. 1–19, doi:10.1007/978-3-319-95489-9_1, ISBN 978-3-319-95489-9, retrieved 2023-12-30
- Ashford's Dictionary of Industrial Chemicals, Third edition, 2011, ISBN 978-0-9522674-3-0, pages 7766-9
- ^ "Product Safety Assessment(PSA): Propylene". Dow Chemical Co. Archived from the original on 2013-08-28. Retrieved 2011-07-11.
- Ghashghaee, Mohammad (2018). "Heterogeneous catalysts for gas-phase conversion of ethylene to higher olefins". Rev. Chem. Eng. 34 (5): 595–655. doi:10.1515/revce-2017-0003. S2CID 103664623.
- Banks, R. L.; Bailey, G. C. (1964). "Olefin Disproportionation. A New Catalytic Process". Industrial & Engineering Chemistry Product Research and Development. 3 (3): 170–173. doi:10.1021/i360011a002.
- Lionel Delaude; Alfred F. Noels (2005). "Metathesis". Kirk-Othmer Encyclopedia of Chemical Technology. Weinheim: Wiley-VCH. doi:10.1002/0471238961.metanoel.a01. ISBN 978-0-471-23896-6.
- Schiffer, Zachary J.; Manthiram, Karthish (2017). "Electrification and Decarbonization of the Chemical Industry". Joule. 1 (1): 10–14. Bibcode:2017Joule...1...10S. doi:10.1016/j.joule.2017.07.008. hdl:1721.1/124019. S2CID 117360588.
- Amghizar, Ismaël; Vandewalle, Laurien A.; Van Geem, Kevin M.; Marin, Guy B. (2017). "New Trends in Olefin Production". Engineering. 3 (2): 171–178. Bibcode:2017Engin...3..171A. doi:10.1016/J.ENG.2017.02.006.
- de Guzman, Doris (October 12, 2012). "Global Bioenergies in bio-propylene". Green Chemicals Blog.
- Wu, Tianwei; Yu, Qingbo; Roghair; et al. (2020). "Chemical looping oxidative dehydrogenation of propane: A comparative study of Ga-based, Mo-based, V-based oxygen carriers". Chemical Engineering and Processing - Process Intensification. 157: 108137. Bibcode:2020CEPPI.15708137W. doi:10.1016/j.cep.2020.108137. ISSN 0255-2701.
- ^ "Market Study: Propylene (2nd edition), Ceresana, December 2014". ceresana.com. Retrieved 2015-02-03.
- Budavari, Susan, ed. (1996). "8034. Propylene". The Merck Index, Twelfth Edition. New Jersey: Merck & Co. pp. 1348–1349.
- J.G.L., Fierro (Ed.) (2006). Metal Oxides, Chemistry and Applications. CRC Press. pp. 414–455.
- For example, "MAPP-Pro"
- Heggs, T. Geoffrey (2011-10-15), "Polypropylene", in Wiley-VCH Verlag GmbH & Co. KGaA (ed.), Ullmann's Encyclopedia of Industrial Chemistry, Weinheim, Germany: Wiley-VCH Verlag GmbH & Co. KGaA, pp. o21_o04, doi:10.1002/14356007.o21_o04, ISBN 978-3-527-30673-2, retrieved 2021-07-09
- Olivier-Bourbigou, H.; Breuil, P. A. R.; Magna, L.; Michel, T.; Espada Pastor, M. Fernandez; Delcroix, D. (2020). "Nickel Catalyzed Olefin Oligomerization and Dimerization" (PDF). Chemical Reviews. 120 (15): 7919–7983. doi:10.1021/acs.chemrev.0c00076. PMID 32786672. S2CID 221124789.
- PubChem. "Hazardous Substances Data Bank (HSDB): 175". pubchem.ncbi.nlm.nih.gov. Retrieved 2021-07-09.
- Bio-based drop-in, smart drop-in and dedicated chemicals
- Duurzame bioplastics op basis van hernieuwbare grondstoffen
- Guzman, Doris de (12 October 2012). "Global Bioenergies in bio-propylene". Green Chemicals Blog. Retrieved 2021-07-09.
- Encyclopedia of Chemical Technology, Fourth edition, 1996, ISBN 0471-52689-4 (v.20), page 261
- Marcelino, N.; Cernicharo, J.; Agúndez, M.; et al. (2007-08-10). "Discovery of Interstellar Propylene (CH2CHCH3): Missing Links in Interstellar Gas-Phase Chemistry". The Astrophysical Journal. 665 (2). IOP: L127–L130. arXiv:0707.1308. Bibcode:2007ApJ...665L.127M. doi:10.1086/521398. S2CID 15832967.
- "Spacecraft finds propylene on Saturn moon, Titan". UPI.com. 2013-09-30. Retrieved 2013-11-12.
- "Cassini finds ingredient of household plastic on Saturn moon". Spacedaily.com. Retrieved 2013-11-12.
GABAA receptor positive modulators | |
---|---|
Alcohols | |
Barbiturates |
|
Benzodiazepines |
|
Carbamates | |
Flavonoids |
|
Imidazoles | |
Kava constituents | |
Monoureides | |
Neuroactive steroids |
|
Nonbenzodiazepines | |
Phenols | |
Piperidinediones | |
Pyrazolopyridines | |
Quinazolinones | |
Volatiles/gases |
|
Others/unsorted |
|
See also: Receptor/signaling modulators • GABA receptor modulators • GABA metabolism/transport modulators |
Binary compounds of hydrogen | |||||||||||||||||||
---|---|---|---|---|---|---|---|---|---|---|---|---|---|---|---|---|---|---|---|
Alkali metal (Group 1) hydrides | |||||||||||||||||||
Alkaline (Group 2) earth hydrides |
| ||||||||||||||||||
Group 13 hydrides |
| ||||||||||||||||||
Group 14 hydrides |
| ||||||||||||||||||
Pnictogen (Group 15) hydrides |
| ||||||||||||||||||
Hydrogen chalcogenides (Group 16 hydrides) |
| ||||||||||||||||||
Hydrogen halides (Group 17 hydrides) |
| ||||||||||||||||||
Transition metal hydrides | |||||||||||||||||||
Lanthanide hydrides | |||||||||||||||||||
Actinide hydrides | |||||||||||||||||||
Exotic matter hydrides |