![]() | This article may require cleanup to meet Misplaced Pages's quality standards. The specific problem is: the article is overly detailed on a single subtopic (75% text on small molecule, preclinical drug discovery), largely based on primary lit, and so its selection and summary are OR. Please help improve this article if you can. (December 2014) (Learn how and when to remove this message) |
CCR5 receptor antagonists are a class of small molecules that antagonize the CCR5 receptor. The C-C motif chemokine receptor CCR5 is involved in the process by which HIV, the virus that causes AIDS, enters cells. Hence antagonists of this receptor are entry inhibitors and have potential therapeutic applications in the treatment of HIV infections.
The life cycle of the HIV presents potential targets for drug therapy, one of them being the viral entry pathway. CCR5 and CXCR4 are the main receptors involved in the HIV entry process. These receptors belong to the seven transmembrane G-protein-coupled receptor (GPCR) family and are predominantly expressed on human T-cells, dendritic cells and macrophages, Langerhans cells. They play an important role as co-receptors that HIV type 1 (HIV-1) uses to attach to cells before viral fusion and entry into host cells. HIV isolates can be divided into R5 and X4 strains. R5 strain is when the virus uses the co-receptor CCR5 and X4 strain is when it uses CXCR4. The location of CCR5 receptors at the cell surface, both large and small molecules have the potential to interfere with the CCR5-viral interaction and inhibit viral entry into human cells.
History
Since the discovery of HIV in the 1980s, remarkable progress has been made in the development of novel antiviral drugs. The trigger for the discovery of the CCR5 antagonists was the observation that a small percentage of high-risk populations showed either resistance or delayed development of the disease. This population was found to have a mutation (CCR5-Δ32) in the gene that codes for the CCR5 receptor which results in almost complete resistance against HIV-1 infection and scientists then discovered the key role of the cell surface receptors CCR5 and CXCR4 in successful viral fusion and infection. In 1996, it was demonstrated that CCR5 serves as a co-receptor for the most commonly transmitted HIV-1 strains, R5. This type of virus is predominant during the early stages of infection and remains the dominant form in over 50% of late stage HIV-1 infected patients, however R5 strains can eventually evolve into X4 as the disease progresses. This information led to the development of a new class of HIV drugs called CCR5 antagonists.
Mechanism of action

HIV enters host cells in the blood by attaching itself to receptors on the surface of the CD4+ cell. Viral entry to the CD4+ cell begins with attachment of the R5 HIV-1 glycoprotein 120 (gp120) to the CD4+ T-cell receptor, which produces a conformational change in gp120 and allows it to bind to CCR5, thereby triggering glycoprotein 41 (gp41) mediated fusion of the viral envelope with the cell membrane and the nucleocapsid enters the host cell (Figure 1). CCR5 co-receptor antagonists prevent HIV-1 from entering and infecting immune cells by blocking CCR5 cell-surface receptor. Small molecule antagonists of CCR5 bind to a hydrophobic pocket formed by the transmembrane helices of the CCR5 receptor. They are thought to interact with the receptor in an allosteric manner locking the receptor in a conformation that prohibits its co-receptor function.
Drug development
As mentioned, the CCR5 receptor is a G-protein coupled receptor (GPCR). Before the discovery of CCR5's role in HIV infection, many pharmaceutical companies had already built a substantial collection of compounds that target GPCRs. Some of these compounds would prove to be a starting point for CCR5 antagonist medicinal chemistry, but would need optimization to improve CCR5 selectivity and potency, and to improve pharmacokinetic properties. A significant problem was the affinity of available screening hits for the hERG ion channel; inhibition of hERG leads to QT interval prolongation, which can increase the risk of developing fatal ventricular arrhythmias. Many CCR5 antagonists have been studied by pharmaceutical companies, but few of them have actually reached human efficacy studies; for example AstraZeneca, Novartis, Merck, and Takeda have used their GPRC-targeting compound collections to develop a potent CCR5 antagonist, but none of them have reached clinical trials. Three pharmaceutical companies were in competition to be the first to have a small molecule CCR5 antagonist approved: GlaxoSmithKline (GSK) with their compound aplaviroc, Schering-Plough with vicriviroc, and Pfizer with maraviroc. All of the compounds reached clinical trials in humans; only maraviroc has been approved by the U.S. Food and Drug Administration (FDA).
Leronlimab
Leronlimab is a humanized monoclonal antibody targeted against the CCR5 receptor found on T lymphocytes of the human immune system and many types of cancers. It is being investigated as a potential therapy in the treatment of HIV infection, graft versus host disease (NCT02737306) and metastatic cancer (NCT03838367). The United States Food and Drug Administration (FDA) has designated leronlimab for fast-track approval. In February 2008, the drug entered Phase 2 clinical trials and a phase 3 trial was begun in 2015. In February 2018 CytoDyn Inc reported that the primary endpoint has been achieved in the PRO 140 pivotal combination therapy trial in HIV infection.
Leronlimab is being developed by CytoDyn Inc. In May 2007, results from the phase I clinical trial of the drug demonstrated "potent, rapid, prolonged, dose-dependent, highly significant antiviral activity" for leronlimab. Participants in the highest-dosing group received 5 milligrams per kilogram and showed an average viral load decrease of -1.83 log10. On average, reductions of greater than -1 log10 per millilitre were maintained for between two and three weeks, from only a single dose of the drug. The largest individual HIV RNA reductions ranged up to -2.5 log10 among patients receiving both the 2 and 5 mg/kg doses.
Leronlimab is a lab-made antibody that functions as an entry inhibitor. Leronlimab binds to the CCR5 receptor on the CD4 cells, and interferes with HIV's ability to enter the cell. Leronlimab, a humanized form of a PA14 antibody, is a chemokine-receptor CCR5 monoclonal antibody and can inhibit CCR5 tropic HIV-1 at concentrations that do not antagonize the natural activity of CCR5 in vitro. HIV-1 entry is mediated by the HIV-1 envelope glycoproteins gp120 and gp41. The gp120 will bind CD4 and the CCR5co receptor molecule, and this triggers gp41-mediated fusion of the viral and cellular membranes. CCR5 is hence needed for the entry of the virus and this infection of healthy cells. Leronlimab, the anti-CCR5 monoclonal antibody, can stop HIV from entering the cell and stop viral replication. It prevents the virus-cell binding at a distinct site in the CCR5 co-receptor without interfering with its natural activity. Unlike other entry inhibitors, PRO 140 is a monoclonal antibody. The mechanism of inhibition is competitive rather than allosteric. As such, it must be injected to be effective. However, once inside the body, PRO 140 binds to CCR5 for >60 days, which may allow for dosing as infrequently as every other week. Compared to highly-active antiretroviral therapy which has been shown to have treatment-related toxicities for HIV-infected patients, PRO140 has no multi-drug resistance or toxicities.
In February 2018, CytoDyn reported that the primary endpoint has been achieved in the PRO 140 pivotal combination therapy trial in HIV infection and will continue for an additional 24 weeks (end of August 2018) with PRO 140 weekly subcutaneous injections and optimized ART. The report discloses that a single 350 mg subcutaneous injection of PRO 140 resulted in a HIV-1 RNA viral load reduction greater than 0.5log or 68% within one week compared with those who received a placebo. The primary efficacy endpoint results were presented at ASM Microbe 2018. In the pivotal trial of leronlimab in combination with standard anti-retroviral therapies in HIV-infected treatment-experienced patients, 81% of patients completing trial achieved HIV viral load suppression of < 50 cp/mL. Recent approved drugs for this population range from 43% after 24 weeks to 45% after 48 weeks with viral load suppression of < 50 cp/mL. In March 2019, CytoDyn filed with the US FDA the first part of the BLA for leronlimab (PRO140) as a combination therapy with HAART in HIV. In May 2020, the company filed its BLA with potential FDA approval in 4Q'20. CytoDyn is conducting an investigative monotherapy trial of leronlimab (PRO140) for HIV. If successful, once per week self-administered leronlimab would represent a paradigm shift in treatment of HIV.
CytoDyn is investigating the use of leronlimab in various solid tumors. On February 18, 2019, CytoDyn announced it will begin 8 pre-clinical studies on melanoma cancer, pancreatic, breast, prostate, colon, lung, liver, and stomach cancer. This has the potential to lead to 8 phase II clinical studies with leronlimab in the cancer arena. On November 23, 2018, CytoDyn received FDA approval of its IND submission and allowed to initiate a Phase Ib/II clinical trial for metastatic triple-negative breast cancer (mTNBC) patients. On February 20, 2019, CytoDyn announced that leronlimab was able to reduce by more than 98% the incidence of human breast cancer metastasis in a mouse xenograft model for cancer through six weeks with leronlimab. The temporal equivalency of the murine 6 weeks study may be up to 6 years in humans. In May 2019, the U.S. Food and Drug Administration (FDA) granted fast track designation for leronlimab for use in combination with carboplatin for the treatment of patients with CCR5-positive mTNBC. In July 2019, CytoDyn announced the dosing of first mTNBC patient under compassionate use. Simultaneously, the Phase Ib/II trial for treatment-naïve mTNBC patients is active and anticipates top line data in 2020. If successful, the data from treatment-naïve mTNBC patients could serve as the basis for potentially seeking accelerated US FDA approval.
A study demonstrated leronlimab reduced the number and size of new human breast cancer metastasis in a mouse model and reduced the size of established metastasis thereby extending survival.
In May 2019, CytoDyn initiated pre-clinical study of leronlimab to prevent NASH.
Aplaviroc


Aplaviroc is originated from a class of spirodiketopiperazine derivatives. Figure 2 shows the molecular structure of the lead compound and the final compound aplaviroc. The lead compound showed good potency in blocking CCR5 in a number of R5 HIV strains and against multi-drug resistant strains. The problem with this compound was not its CCR5 selectivity but the oral bioavailability. This led to further development of the molecule and the result was a compound named aplaviroc. Unfortunately, despite the promising preclinical and early clinical results, some severe liver toxicity was observed in the treatment of naïve and treatment-experienced patients that led to the discontinuation in further development of aplaviroc.
Vicriviroc
Schering-Plough identified an active compound during screening. Figure 3 shows the molecular structure of the lead compound, intermediate compound, and the final compound vicriviroc. The lead compound contained a piperazine scaffold and was a potent muscarinic acetylcholine receptor (M2) antagonist with modest CCR5 activity. The changes that were made on the left hand side of the lead compound and the addition of a methyl group on the piperazine group ((S)-methylpiperazine) resulted in the intermediate compound that had good affinity for CCR5 receptors but very little affinity for muscarinic activity, however, the compound did show affinity for the hERG ion channel. Further reconstruction led to the development of the final compound vicriviroc, when Schering discovered that the pyridyl N-oxide on the intermediate could be replaced by 4,6-dimethylpyrimidine carboxamide. Vicriviroc had an excellent selectivity for CCR5 receptors over muscarinic and hERG affinity was greatly reduced. Phase I clinical trial of vicriviroc gave promising results, so a phase II study in the treatment of naïve patients was initiated. The phase II study was discontinued since there was a viral breakthrough in the vicriviroc group compared to the control group. These results suggested that vicriviroc was not effective in the treatment of treatment-naïve patients compared to current treatments. Another phase II clinical study was performed in treatment-experienced patients. The results were that vicriviroc did have strong antiviral activity but five instances of cancer among the participants were reported, however, the study was continued since there was lack of causal association of the malignancies and vicriviroc. In late 2009, vicriviroc was reported by the company to have entered phase II studies in treatment for naïve patients and phase III studies in treatment-experienced patients.
Maraviroc
Pfizer turned to high-throughput screening in their search for a good starting point for a small molecule CCR5 antagonist. Their screening resulted in a compound that presented weak affinity and no antiviral activity but represented a good starting point for further optimization. Compounds 1–9 in Table 1 show the development of maraviroc in few steps. The chemical structure of the starting molecule (UK-107,543) is presented as compound 1. Their first focus was to minimize CYP2D6 activity of the molecule and to reduce its lipophilicity. They replaced the imidazopyridine with benzimidazole and the benzhydril group was swapped out for a benzamide. The outcome was compound 2. That compound showed good binding potency and the start of an antiviral activity. Further structure–activity relationship (SAR) optimization of the amide region and identifying the enantiomeric preference led to the cyclobutyl amide structure in compound 3. However, the problem with the CYP2D6 activity of the compound was still unacceptable so they had to perform further SAR optimization that determined that the -azabicycloamine (tropane) could replace the aminopiperidine moiety. This change in the chemical structure led to compound 4. Compound 4 had no CYP2D6 activity while preserving excellent binding affinity and antiviral activity. Although compound 4 showed promising results, it demonstrated 99% inhibition on the hERG ion channel. That inhibition was unacceptable since it can lead to QTc interval prolongation. The research team then did a few modifications to see which part of the molecule played a role in the hERG affinity. Compound 5 shows an analogue that they synthesized which contained an oxygen bridgehead in the tropane ring; however, that reconstruction did not have an effect on the hERG affinity. They then focused on the polar surface area in the molecule to dial out the hERG affinity. These efforts resulted in compound 6. That compound preserved desired antiviral activity and was selective against the hERG inhibition but the problem was its bioavailability. Reduction in the lipophilicity, by replacing the benzimidazol group with a substituted triazole group gave compound 7. Compound 7 had shown a significant reduction in lipophilicity and maintained the antiviral activity but again, with the introduction of a cyclobutyl group, the compound showed hERG inhibition. Changing the ring size in compound 7 from a cyclobutyl unit to a cyclopentyl unit in compound 8 led to a significant increase in antiviral activity and loss of hERG affinity. Further development led to discovery of a 4,4'-difluorocyclohexylamide also known as maraviroc. Maraviroc preserved excellent antiviral activity, whilst demonstrating no significant hERG binding affinity. The lack of hERG binding affinity was predicted to be because of the large size of the cyclohexyl group and the high polarity of the fluoro substituents. In August 2007 the FDA approved the first CCR5 antagonist, maraviroc, discovered and developed by Pfizer.
Pharmacophore

The predictive pharmacophore model was developed for a large series of piperidine- and piperazine-based CCR5 antagonists by Schering-Plough Research Institute. Their hypothesis consisted of mostly five features, two hydrogen bond acceptors, marked C and D in figure 4 and three hydrophobic groups, A, B and E in figure 4. Part B usually has a basic nitrogen group. The model was validated using diverse set of six CCR5 antagonists from five different pharmaceutical companies. The best model correctly predicted these compounds as being highly active. It is possible to use the model as a tool in virtual screening for new small molecular CCR5 antagonists and also to predict biological activities of compounds prior to undertaking their costly synthesis.
Binding
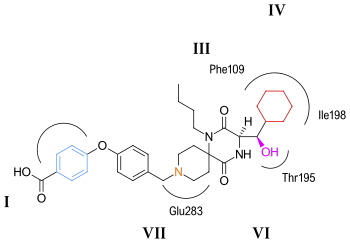

CCR5 is a member of G protein-coupled, seven transmembrane segment receptors. The structure of the receptor comprises seven-helix bundle in the transmembrane region, these regions are labeled I–VII in figures 5 and 6. The CCR5 antagonists are predicted to bind to a putative binding pocket which is buried inside the transmembrane domain, enclosed by the seven transmembrane helices. The binding pocket is very hydrophobic with multiple aromatic residues lining the pocket. The key residues are tryptophan 86 and 248 (Trp86, Trp248), tyrosine 108 and 251 (Tyr108, Tyr251), phenylalanine 109 (Phe109), threonine 195 (Thr195), isoleucine 198 (Ile198), glutamic acid 283 (Glu283). CCR5 antagonists are very different in shape and electrostatic potential although they all share the same binding pocket. The interesting thing about the binding of these molecules is that they exhibit significantly different binding modes, although they all establish an extensive interaction network with CCR5.
Aplaviroc
The putative binding mode for aplaviroc is shown in figure 5. The key saltbridge interaction between aplaviroc and Glu283 is predicted to be quite weak compared to other CCR5 antagonists. The hydroxyl group on aplaviroc forms a strong hydrogen bond to the polar residue Thr195. This H-bond interaction is the strongest with aplaviroc compared to other CCR5 antagonists. The cyclohexyl group in the aplaviroc structure is predicted to interact with the receptor in a hydrophobic pocket formed by Ile198, Thr195 and Phe109 and is thought to show quite strong hydrophobic interactions. The researchers predict that the butyl group of aplaviroc is buried within the helical bundle through strong hydrophobic interaction with multiple aromatic residues of the CCR5 receptor. Aplaviroc has a unique feature of preserving two of the natural chemokine protein ligands binding to CCR5 and subsequent activation, whereas maraviroc and the other antagonists almost fully block chemokine-CCR5 interactions. This kind of interference is so far considered to be safe, and individuals that naturally lack CCR5 do not show any obvious health problems. However, to limit the toxicity and side effects of CCR5 antagonists it would be ideal to be able to preserve the chemokine receptor function. Consequently, it should be of interest to design inhibitors that specifically disrupt CCR5–gp120 binding but do not affect the CCR5 chemokine activation.
Maraviroc
The putative binding mode for maraviroc is shown in figure 6. The strongest interaction is estimated to be between maraviroc and glutamic acid (Glu283) through a strong salt bridge interaction. The interaction between tryptophan (Trp86) and maraviroc involves T-shaped π-π stacking while the interaction with phenylalanine (Phe109) is predicted to be hydrophobic. Tyrosine (Tyr108) is thought to interact with the phenyl group on maraviroc through a parallel displaced interaction. The interaction between maraviroc and isoleucine (Ile198) is predicted to be mostly hydrophobic in nature and the interaction between maraviroc and tyrosine (Tyr251) is very limited.
Other CCR5 antagonists

Development of new CCR5 antagonists continues, both for their antiviral effects and also for potential utility in a variety of autoimmune indications. Researchers at Roche Palo Alto discovered a novel series of potent CCR5 small-molecule antagonists. Lead optimization was pursued by balancing opposing trends of metabolic stability and potency. Combination of the spiropiperidine template with pharmacophore elements from both aplaviroc, and Schering's CCR5 antagonist program, led to the initial lead compound in this series. Further development of that lead compound led to the discovery of compound A in figure 7 — a compound that possesses a good selectivity and pharmacokinetic properties.
The CCR5 antagonist INCB009471 has nanomolar activity against HIV-1 in vitro. This compound demonstrated potent and prolonged antiviral activity against R5-tropic HIV-1 when given 200 mg once daily dose for 14 days. These findings supported further clinical development of INCB009471 and they have since progressed to phase IIb clinical trials. As of 2009 the study of this compound is inactive and no further studies are planned at this time.
Not only small molecules but also proteins delivered by gene therapy have been suggested to ablate CCR5 function, an approach that has also been employed for other HIV targets.
See also
- HIV/AIDS research
- Cenicriviroc
- CD4
- CCL5
- CCR5
- Subtypes of HIV
- HIV tropism
- Discovery and development of non-nucleoside reverse transcriptase inhibitors
- Discovery and development of nucleoside and nucleotide reverse-transcriptase inhibitors
References
- ^ Lederman MM, Penn-Nicholson A, Cho M, Mosier D (August 2006). "Biology of CCR5 and its role in HIV infection and treatment". JAMA. 296 (7): 815–26. doi:10.1001/jama.296.7.815. PMID 16905787.
- ^ De Clercq E (December 2007). "The design of drugs for HIV and HCV". Nature Reviews Drug Discovery. 6 (12): 1001–18. doi:10.1038/nrd2424. PMID 18049474. S2CID 37859193.
- ^ Pulley, Shon (2007). "CCR5 antagonists: from discovery to clinical efficacy". In Neote, Kuldeep; Letts, Gordon L.; Moser, Bernhard (eds.). Chemokine Biology — Basic Research and Clinical Application. Progress in Inflammation Research. Vol. 2. Birkhauser Basel. pp. 145–163. doi:10.1007/978-3-7643-7437-2_11. ISBN 978-3-7643-7195-1.
- ^ Lalezari, J.; Goodrich, J.; DeJesus, E.; Lampiris, H.; Gulick, R.; Saag, M.; Ridgway, C.; McHale, M.; Van Der Ryst, E. "Efficacy and Safety of Maraviroc plus Optimized Background Therapy In Viremic, ART-Experienced Patients Infected With CCR5-Tropic HIV‑1, 24-week results of a Phase 2b/3 study in the U. S. & Canada. Abstract 104bLB". 14th Conference on Retroviruses and Opportunistic Infections. Archived from the original on 2009-03-18. Retrieved 2009-11-03.
- Samson M, Libert F, Doranz BJ, et al. (August 1996). "Resistance to HIV-1 infection in caucasian individuals bearing mutant alleles of the CCR-5 chemokine receptor gene". Nature. 382 (6593): 722–5. Bibcode:1996Natur.382..722S. doi:10.1038/382722a0. PMID 8751444. S2CID 4370818.
- Dragic T, Litwin V, Allaway GP, et al. (June 1996). "HIV-1 entry into CD4+ cells is mediated by the chemokine receptor CC-CKR-5". Nature. 381 (6584): 667–73. Bibcode:1996Natur.381..667D. doi:10.1038/381667a0. PMID 8649512. S2CID 4301976.
- ^ Flexner C (December 2007). "HIV drug development: the next 25 years". Nature Reviews Drug Discovery. 6 (12): 959–66. doi:10.1038/nrd2336. PMID 17932493. S2CID 31261997.
- ^ Ray N, Doms RW (2006). "HIV-1 Coreceptors and Their Inhibitors". Chemokines and Viral Infection. Current Topics in Microbiology and Immunology. Vol. 303. pp. 97–120. doi:10.1007/978-3-540-33397-5_5. ISBN 978-3-540-29207-4. PMID 16570858.
- Westby M, van der Ryst E (2005). "CCR5 antagonists: host-targeted antivirals for the treatment of HIV infection". Antiviral Chemistry & Chemotherapy. 16 (6): 339–54. doi:10.1177/095632020501600601. PMID 16329283. S2CID 24994759.
- Briz V, Poveda E, Soriano V (April 2006). "HIV entry inhibitors: mechanisms of action and resistance pathways". The Journal of Antimicrobial Chemotherapy. 57 (4): 619–27. doi:10.1093/jac/dkl027. PMID 16464888.
- Murga JD, Franti M, Pevear DC, Maddon PJ, Olson WC (October 2006). "Potent Antiviral Synergy between Monoclonal Antibody and Small-Molecule CCR5 Inhibitors of Human Immunodeficiency Virus Type 1". Antimicrobial Agents and Chemotherapy. 50 (10): 3289–96. doi:10.1128/AAC.00699-06. PMC 1610098. PMID 17005807.
- Watson C, Jenkinson S, Kazmierski W, Kenakin T (April 2005). "The CCR5 receptor-based mechanism of action of 873140, a potent allosteric noncompetitive HIV entry inhibitor". Molecular Pharmacology. 67 (4): 1268–82. doi:10.1124/mol.104.008565. PMID 15644495. S2CID 2587200.
- Fermini B, Fossa AA (June 2003). "The impact of drug-induced QT interval prolongation on drug discovery and development". Nature Reviews Drug Discovery. 2 (6): 439–47. doi:10.1038/nrd1108. PMID 12776219. S2CID 27492332.
- Cumming JG, Cooper AE, Grime K, et al. (November 2005). "Modulators of the human CCR5 receptor. Part 2: SAR of substituted 1-(3,3-diphenylpropyl)-piperidinyl phenylacetamides". Bioorganic & Medicinal Chemistry Letters. 15 (22): 5012–5. doi:10.1016/j.bmcl.2005.08.014. PMID 16154744.
- Thoma G, Nuninger F, Schaefer M, Akyel KG, Albert R, Beerli C, Bruns C, Francotte E, Luyten M, MacKenzie D, Oberer L, Streiff MB, Wagner T, Walter H, Weckbecker G, Zerwes HG (April 2004). "Orally bioavailable competitive CCR5 antagonists". Journal of Medicinal Chemistry. 47 (8): 1939–55. doi:10.1021/jm031046g. PMID 15055994.
- Bryan Oates; Richard J. Budhu; Sander G. Mills; Malcolm MacCoss; Lorraine Malkowitz; Martin S. Springer; Bruce L. Daugherty; Sandra L. Gould; Julie A. DeMartino; Salvatore J. Siciliano; Anthony Carella; Gwen Carver; Karen Holmes; Renee Danzeisen; Daria Hazuda; Joseph Kessler; Janet Lineberger; Michael Miller; William A. Schleif; Emilio A. Emini (January 2001). "Antagonists of the human CCR5 receptor as anti-HIV-1 agents. Part 1: discovery and initial structure-activity relationships for 1 -amino-2-phenyl-4-(piperidin-1-yl)butanes". Bioorganic & Medicinal Chemistry Letters. 11 (2): 259–64. doi:10.1016/S0960-894X(00)00637-5. PMID 11206473.
- Tremblay CL, Giguel F, Guan Y, Chou TC, Takashima K, Hirsch MS (August 2005). "TAK-220, a Novel Small-Molecule CCR5 Antagonist, Has Favorable Anti-Human Immunodeficiency Virus Interactions with Other Antiretrovirals In Vitro". Antimicrobial Agents and Chemotherapy. 49 (8): 3483–5. doi:10.1128/AAC.49.8.3483-3485.2005. PMC 1196290. PMID 16048964.
- "CytoDyn Announces Acquisition of PRO 140" (Press release). CytoDyn Inc. October 17, 2012. Archived from the original on September 25, 2013. Retrieved September 3, 2019.
- Brian Lawler. Progenics' Intriguing Study Results. The Motley Fool. 2 May 2007.
- "Phase 2 clinical trials started on PRO 140". AIDS Patient Care and STDs. 22 (2): 159–60. February 2008. doi:10.1089/apc.2008.9960. PMID 18273941.
- "Cytodyn Initiates First Clinical Site for Phase 3 Trial of PRO 140". CytoDyn (Press release). Retrieved 2015-08-22.
- "CytoDyn Reports Primary Endpoint Achieved in PRO 140 Pivotal Combination Therapy Trial in HIV Infection". CytoDyn (Press release). Retrieved 2018-04-19.
- Derek Thaczuk. ICAAC: Phase 1 study provides 'proof of concept' for PRO 140, a monoclonal CCR5 antibody Archived 2007-10-27 at the Wayback Machine. AIDSmap.com. 21 Sept 2007.
- Liz Highleyman. Monoclonal Antibody CCR5 Inhibitor PRO 140 Produces Long-lasting HIV Suppression in Single-dose Study. 28 Sept 2007. HIVandHepatitis.com
- Biswas P, Tambussi G, Lazzarin A (2007). "Access denied? The status of co-receptor inhibition to counter HIV entry". Expert Opin Pharmacother. 8 (7): 923–33. doi:10.1517/14656566.8.7.923. PMID 17472538. S2CID 32675897.
- Pugach P, Ketas TJ, Michael E, Moore JP (August 2008). "Neutralizing antibody and anti-retroviral drug sensitivities of HIV-1 isolates resistant to small molecule CCR5 inhibitors". Virology. 377 (2): 401–7. doi:10.1016/j.virol.2008.04.032. PMC 2528836. PMID 18519143.
- ^ L, Li, Sun T, Yang K, Zhang P, and Jia WQ. "Monoclonal CCR5 Antibody for Treatment of People with HIV Infection (Review)." Cochrane Database of Systematic Reviews 2 (2011): n. pag. Print.
- "Progenics Pharmaceuticals' HIV Drug, PRO 140, Receives FDA Fast-Track Designation" Archived 2011-10-02 at the Wayback Machine. Press release, 22 February 2006. Progenics Pharmaceuticals. progenics.com.
- "PRO 140" Archived 2012-04-02 at the Wayback Machine. Progenics Pharmaceuticals. progenics.com.
- Tim Horn. "Single-Dose PRO 140 Has Lasting Effects". 21 Sept 2007. POZ.com.
- "CytoDyn Reports Primary Endpoint Achieved in PRO 140 Pivotal Combination Therapy Trial in HIV Infection". CytoDyn (Press release). Retrieved 2018-04-19.
- "CytoDyn to Present Primary Efficacy Endpoint Results from its PRO 140 Pivotal Trial in Late-Breaking Session at ASM Microbe 2018". CytoDyn (Press release). Retrieved 2018-04-19.
- "Primary Efficacy Results of PRO 140 SC in a Pivotal Phase 2b/3 Study in Heavily Treatment-Experienced HIV-1 Patients". www.abstractsonline.com. Retrieved 2018-04-19.
- Jiao X, Wang M, Zhang Z, Li Z, Ni D, Ashton AW, Tang HY, Speicher DW, Pestell RG, et al. (January 2021). "Leronlimab, a humanized monoclonal antibody to CCR5, blocks breast cancer cellular metastasis and enhances cell death induced by DNA damaging chemotherapy". Breast Cancer Research. 23 (1): 11. doi:10.1186/s13058-021-01391-1. PMC 7825185. PMID 33485378.
- Maeda K, Nakata H, Koh Y, et al. (August 2004). "Spirodiketopiperazine-Based CCR5 Inhibitor Which Preserves CC-Chemokine/CCR5 Interactions and Exerts Potent Activity against R5 Human Immunodeficiency Virus Type 1 In Vitro". Journal of Virology. 78 (16): 8654–62. doi:10.1128/JVI.78.16.8654-8662.2004. PMC 479103. PMID 15280474.
- Tagat JR, McCombie SW, Steensma RW, et al. (August 2001). "Piperazine-based CCR5 antagonists as HIV-1 inhibitors. I: 2(S)-methyl piperazine as a key pharmacophore element". Bioorganic & Medicinal Chemistry Letters. 11 (16): 2143–6. doi:10.1016/S0960-894X(01)00381-X. PMID 11514156.
- Tagat JR, Steensma RW, McCombie SW, et al. (October 2001). "Piperazine-based CCR5 antagonists as HIV-1 inhibitors. II. Discovery of 1--4- methyl-4-ethyl]-1-piperazinyl]- piperidine N1-oxide (Sch-350634), an orally bioavailable, potent CCR5 antagonist". Journal of Medicinal Chemistry. 44 (21): 3343–6. doi:10.1021/jm0155401. PMID 11585438.
- McCombie SW, Tagat JR, Vice SF, et al. (February 2003). "Piperazine-based CCR5 antagonists as HIV-1 inhibitors. III: synthesis, antiviral and pharmacokinetic profiles of symmetrical heteroaryl carboxamides". Bioorganic & Medicinal Chemistry Letters. 13 (3): 567–71. doi:10.1016/S0960-894X(02)00918-6. PMID 12565973.
- Tagat JR, McCombie SW, Nazareno D, et al. (May 2004). "Piperazine-based CCR5 antagonists as HIV-1 inhibitors. IV. Discovery of 1-- 4-ethyl-3(S)-methyl-1-piperazinyl]- 4-methylpiperidine (Sch-417690/Sch-D), a potent, highly selective, and orally bioavailable CCR5 antagonist". Journal of Medicinal Chemistry. 47 (10): 2405–8. doi:10.1021/jm0304515. PMID 15115380.
- "Schering-Plough Reports Long-Term Vicriviroc Data From Phase II Open-Label Extension Study in Treatment-Experienced HIV-Infected Patients" (Press release). Schering-Plough. 14 September 2009. Retrieved 8 November 2009.
- Dorr, P; Westby, M; Dobbs, S; Griffin, P; Irvine, B; Macartney, M; Mori, J; Rickett, G; Smith-Burchnell, C; Napier, C; Webster, R; Armour, D; Price, D; Stammen, B; Wood, A; Perros, M (November 2005). "Maraviroc (UK-427,857), a Potent, Orally Bioavailable, and Selective Small-Molecule Inhibitor of Chemokine Receptor CCR5 with Broad-Spectrum Anti-Human Immunodeficiency Virus Type 1 Activity". Antimicrobial Agents and Chemotherapy. 49 (11): 4721–32. doi:10.1128/AAC.49.11.4721-4732.2005. PMC 1280117. PMID 16251317.
- ^ Wood A, Armour D (2005). The discovery of the CCR5 receptor antagonist, UK-427,857, a new agent for the treatment of HIV infection and AIDS. Progress in Medicinal Chemistry. Vol. 43. pp. 239–71. doi:10.1016/S0079-6468(05)43007-6. ISBN 978-0-444-51572-8. PMID 15850827.
- ^ Price DA, Armour D, de Groot M, et al. (September 2006). "Overcoming HERG affinity in the discovery of the CCR5 antagonist maraviroc". Bioorganic & Medicinal Chemistry Letters. 16 (17): 4633–7. doi:10.1016/j.bmcl.2006.06.012. PMID 16782336.
- Debnath AK (October 2003). "Generation of predictive pharmacophore models for CCR5 antagonists: study with piperidine- and piperazine-based compounds as a new class of HIV-1 entry inhibitors". Journal of Medicinal Chemistry. 46 (21): 4501–15. doi:10.1021/jm030265z. PMID 14521412.
- Maeda K, Das D, Ogata-Aoki H, et al. (May 2006). "Structural and molecular interactions of CCR5 inhibitors with CCR5". The Journal of Biological Chemistry. 281 (18): 12688–98. doi:10.1074/jbc.M512688200. PMID 16476734.
- ^ Kondru R, Zhang J, Ji C, et al. (March 2008). "Molecular interactions of CCR5 with major classes of small-molecule anti-HIV CCR5 antagonists". Molecular Pharmacology. 73 (3): 789–800. doi:10.1124/mol.107.042101. PMID 18096812. S2CID 16267853.
- ^ Wang T, Duan Y (June 2008). "Binding Modes of CCR5-targetting HIV Entry Inhibitors: Partial and Full Antagonists". Journal of Molecular Graphics & Modelling. 26 (8): 1287–95. doi:10.1016/j.jmgm.2007.12.003. PMC 2701198. PMID 18249144.
- Arimont A, Sun S, Smit MJ, Leurs R, de Esch IJ, de Graaf C (2017). "Structural Analysis of Chemokine Receptor-Ligand Interactions". J Med Chem. 60 (12): 4735–4779. doi:10.1021/acs.jmedchem.6b01309. PMC 5483895. PMID 28165741.
- Rotstein DM, Gabriel SD, Makra F, et al. (September 2009). "Spiropiperidine CCR5 antagonists". Bioorganic & Medicinal Chemistry Letters. 19 (18): 5401–6. doi:10.1016/j.bmcl.2009.07.122. PMID 19674898.
- Kuritzkes DR (March 2009). "HIV-1 Entry Inhbitors: An Overview". Current Opinion in HIV and AIDS. 4 (2): 82–7. doi:10.1097/COH.0b013e328322402e. PMC 2753507. PMID 19339945.
- Swan CH, Bühler B, Steinberger P, Tschan MP, Barbas CF 3rd, Torbett BE, et al. (June 2006). "T-cell protection and enrichment through lentiviral CCR5 intrabody gene delivery". Gene Ther. 13 (20): 1480–92. doi:10.1038/sj.gt.3302801. PMID 16738691.
- Zhang C, Ötjengerdes RM, Roewe J, Mejias R, Marschall AL, et al. (April 2020). "Applying Antibodies Inside Cells: Principles and Recent Advances in Neurobiology, Virology and Oncology". BioDrugs. 34 (4): 435–462. doi:10.1007/s40259-020-00419-w. PMC 7391400. PMID 32301049.
External links
Chemokine receptor modulators | |||||||||||||||||||||||||
---|---|---|---|---|---|---|---|---|---|---|---|---|---|---|---|---|---|---|---|---|---|---|---|---|---|
CC |
| ||||||||||||||||||||||||
CXC |
| ||||||||||||||||||||||||
C (XC) |
| ||||||||||||||||||||||||
CX3C |
| ||||||||||||||||||||||||
Others |
|